Geotextile technology treats discharged materials for horticulture systems

Consumer-driven demand for aquaculture products has resulted in the adoption of intensive fish production facilities. Initial efforts to intensify aquaculture operations were linked to water quality degradation because of increased nitrogen and phosphorus concentrations in discharged wastes. New aquaculture facilities can utilize recirculating aquaculture systems (RAS) to manage solid waste and dissolved nutrients in a controlled environment.
An RAS facility allows the producer to raise fish at high density and minimize freshwater exchange through mechanical removal of solids and biological assimilation of dissolved wastes. RAS technology and aquaculture facilities will be important for future agricultural growth and to conserve the quantity and quality of water resources.
RAS waste
To maintain optimum water quality, an RAS facility typically must discharge concentrated waste daily. Aquaculture effluent is primarily comprised of water, feces, uneaten feed, nitrogen and phosphorus. Around one-third of the nitrogen applied to an aquaculture production system is harvested with the target species. Thus, much is unused, and the potential for improved nutrient efficiency through integrated agricultural systems is high.

Aquaponic systems combine fish production with hydroponic plant production in an RAS. Studies at the University of the Virgin Islands found dissolved nutrients from treated Nile tilapia effluent were capable of supporting a variety of hydroponic vegetable crops and suggested treated effluent should be redirected into secondary enterprises that have economic value or in some way complement the primary production system.
In addition, discharged fish effluent has been applied to land. Research has showed aquaculture effluent performed similar to or better than other organic and inorganic fertilizers for soil-based horticulture production systems. Nonetheless, more research is needed to determine methods to utilize the discharged effluent from RAS for plant production.
Biofloc RAS
Biofloc production systems incorporate RAS principles to produce popular food species like Nile tilapia (Oreochromis niloticus) and Pacific white shrimp (Litopenaeus vannemei). Biofloc systems rely on mechanical suspension of organic matter in the water column. Nitrifying bacteria colonize the suspended organic matter and help keep toxic ammonia and nitrite concentrations at safe levels for the target species, but total suspended solids must be controlled within the culture system.
Auburn research
Research at Auburn University sought to determine methods to utilize the discharged aquaculture effluent for plant production. Auburn University has two, 100-m3 biofloc tanks housed in a 9.2- x 29.5-meter greenhouse at the E. W. Shell Fisheries Center at Auburn University in Auburn, Alabama, USA.
Each rectangular tank was stocked with 4,000 tilapia fingerlings with individual weights of about 100 grams. The fish were harvested at a final weight of 550 to 625 g. The tilapia were fed ad libitum with an extruded diet containing 36 percent protein twice daily for 20 minutes. Calcium hydroxide was added to the production unit as needed to maintain pH at 6.8 to 7.0.
Total suspended solids concentrations were controlled using an external 1.9-cubic-meter tank with a 30-degree cone bottom. The tank had a central baffle perpendicular to the incoming water flow that diminished hydraulic velocity, allowing large particulate matter to settle and concentrate in the cone bottom. After exiting the settling tank, treated water returned to the fish culture tanks. Settled solids were discharged from the cone bottom tank twice daily.
Solids flocculation, effluent dewatering
After discharge, the effluent was injected with a polymer to flocculate the solids before entering a geotextile bag constructed from a high-strength, porous polypropylene fabric. The bag was an effective tool for passively dewatering effluent that created two separate components. Total suspended solids were retained within the bag, while a filtrate low in TSS concentration exited.
After repeated fillings to reach its volumetric capacity, the bag was allowed to passively dewater. Solids were removed, allowed to air dry and milled with a hammer mill. The dried solids had a nitrogen:phosphorus:potassium ratio of 3:11:1, making them a good organic-based material for plant production. Substrates were prepared prior to each experiment by mixing a commercial peat- and pine bark-based growing mix (C.M.) and dewatered aquaculture effluent (A.E.) based on the volume of each substrate.
After mixing, it was important to evaluate the physical and chemical properties of each substrate, because they are important factors in plant growth response. Physical properties like air space affect gas exchange in the substrate, while water-holding capacity affects water retention in the substrate. In general, container substrates should provide physical properties with 10 to 30 percent air space and 40 to 50 percent water-holding capacity. Also, the chemical properties of most horticulture substrates should provide pH values of 5.5 to 7.0 and soluble salt levels below 2.5 µS/cm2.
Experiments
The first experiment evaluated tomato seedlings grown in four different substrates partially replaced with dewatered aquaculture effluent. Treatments included a 100 percent C.M. control and substrates with 25, 50 or 75 percent A.E. All plants were watered with municipal water daily and provided 100 mg/L nitrogen twice weekly. Nutrient solution was formulated from a 20-10-20 water-soluble fertilizer.
After 30 days, tomato plant growth parameters decreased in the substrates with 25 percent and greater A.E. The bulk densities of the substrates with these greater amounts of A.E. increased and resulted in decreased air space for plant roots. Plant roots require space for gas exchange, and the decreased air space negatively affected plant growth. The substrates with 25 percent or greater A.E. content had pH values elevated above 7.0 and soluble salts measured above 2.5 µS/cm2.
A second experiment evaluated the growth of tomatoes in five different substrates partially replaced with dewatered aquaculture effluent. For this experiment, treatments included a 100 percent C.M. control and substrates with 5, 10, 15 or 20 percent A.E. All plants were watered with municipal water and provided 100 mg/L nitrogen twice weekly.
After 30 days, plants grown in substrates with 15 percent or lower A.E. grew similar to or better than the control. The physical properties of substrates with 15 percent or less A.E. were sufficient for tomato seedling growth. The chemical properties of substrates with 15 percent or less A.E. maintained optimal pH and soluble salt levels. However, pH and salt levels began to increase in substrate with 20 percent A.E., and plant growth parameters decreased.
Water source
The second experiment demonstrated that for tomato seedling production, partial substitution of commercially available potting mix with dewatered aquaculture effluent had a positive effect on plant growth and quality if used at a 15 percent or lower portion of the mixture. But it didn’t determine if the nutrients in the aquaculture effluent could replace commercial substrate and provide adequate nutrition for tomato transplants, because all plants were provided a complete nutrient solution twice weekly.
A third experiment evaluated tomato seedling growth grown under specific combinations of substrates and water sources. All plants were grown in one of the following substrates: a 100 percent C.M. control or substrates with 5 or 10 percent A.E. Half the plants grown in each substrate were watered only with municipal water for the entirety of the experiment. The other half were additionally given water that contained 100 mg/L nitrogen from a 20-10-20 water-soluble fertilizer twice weekly.
Neither substrate nor water source created sub-optimal physical or chemical parameters for the tomato seedlings. Tomato seedlings watered with fertilizer and grown in substrates with 0 or 5 percent A.E. had better growth than those grown in the same substrates and watered with municipal water only. These potting mixtures alone were unable to supply the plants with an adequate amount of nutrients, but watering the substrates with 100 mg/L nitrogen improved plant growth.
Water source had no significant impact on growth for plants grown in 10 percent A.E., indicating this substrate could provide optimal physical and chemical parameters and sufficient nutrients for tomato plant growth without the need for watering with 100 mg/L nitrogen.
Economic analysis
A basic economic analysis determined the material cost of dewatering solids using the geotextile bag and polymer. It was based on an actual five-month production period with Nile tilapia produced in the 100-m3 biofloc system.
The cost of the geotextile bag was U.S. $171, and two, 19-L biopolymer buckets ($102 each) were required to flocculate the discharged effluent. The total cost of materials was $375.
A total of 1,505 kg of dry-weight diet was fed to the system over the five-month production period. Approximately 284 kg of dry-weight solids, or 19 percent of the diet fed, was discharged and captured in the geotextile bag. This equated to U.S. $1.32/kg of dry-weight solids.
Perspectives
Concentrated aquaculture wastes will have to be treated on site as RAS facilities intensify their production. Geotextile technology can treat the discharged aquaculture effluent and create a solids component for traditional horticulture production systems.
Through a series of experiments, the authors observed that dewatered aquaculture effluent can replace commercial potting mixes and provide tomato seedlings with adequate nutrients for growth. This information could be useful for farms integrating aquaculture and horticulture on site.
(Editor’s Note: This article was originally published in the May/June 2015 print edition of the Global Aquaculture Advocate.)
Authors
-
Jason Danaher, Ph.D.
Aquatic System Design
Pentair Aquatic Eco-Systems, Inc.
2395 Apopka Boulevard
Apopka, Florida 32703 USA[109,111,99,46,114,105,97,116,110,101,112,64,114,101,104,97,110,97,100,46,110,111,115,97,106]
-
Jeremy Pickens
Department of Horticulture
Auburn University
Auburn, Alabama, USA -
Jeffrey Sibley, Ph.D.
Department of Horticulture
Auburn University
Auburn, Alabama, USA -
Jesse Chappell, Ph.D.
School of Fisheries, Aquaculture and Aquatic Sciences
Auburn University
Auburn, Alabama, USA -
Terrill Hanson, Ph.D.
School of Fisheries, Aquaculture and Aquatic Sciences
Auburn University
Auburn, Alabama, USA
Tagged With
Related Posts

Aquafeeds
Biofloc systems viable for tilapia production
Well-designed and managed biofloc technology systems are a viable alternative for production of various species like tilapia, to increase feed efficiency by lowering aquafeed protein requirements and to help reduce or eliminate effluent discharges.

Responsibility
A look at various intensive shrimp farming systems in Asia
The impact of diseases led some Asian shrimp farming countries to develop biofloc and recirculation aquaculture system (RAS) production technologies. Treating incoming water for culture operations and wastewater treatment are biosecurity measures for disease prevention and control.

Responsibility
Can sustainable mariculture match agriculture’s output?
Global, sustainable mariculture production, developed on a massive, sustainable scale and using just a small fraction of the world’s oceanic areas, could eventually match the output of land-based agriculture production. Scale and international law considerations require the involvement of many stakeholders, including national governments and international organizations.
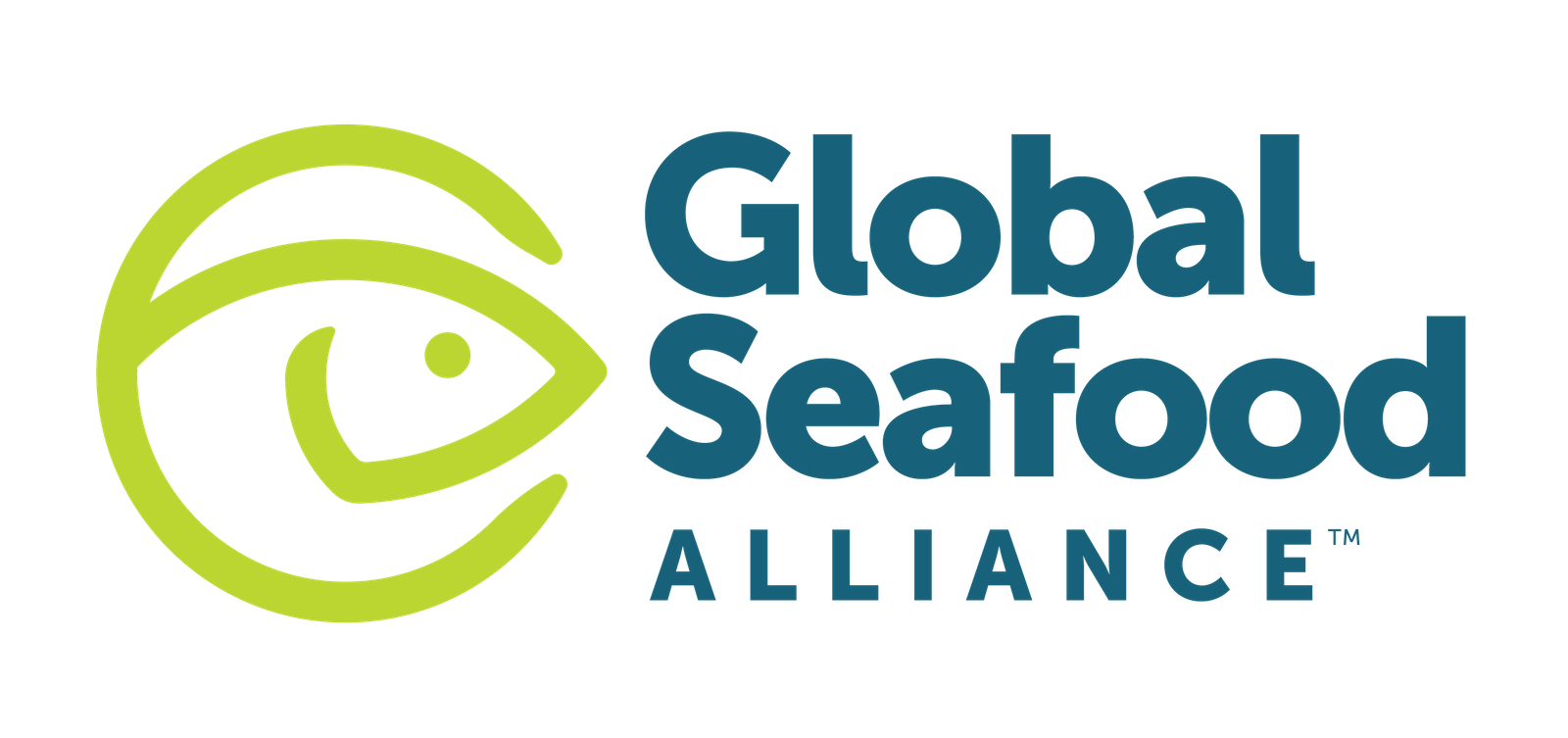
Intelligence
An engineer’s design for a classroom aquaculture-aquaponics system
An aquaponics teaching system was designed, built and operated by students at the University of Arizona, integrating its operation and management into the educational curriculum. This engineering design will require minimum maintenance and will last years.