Brazilian researchers recommend 12.5:1 ratio for mixed heterotrophic and autotrophic systems
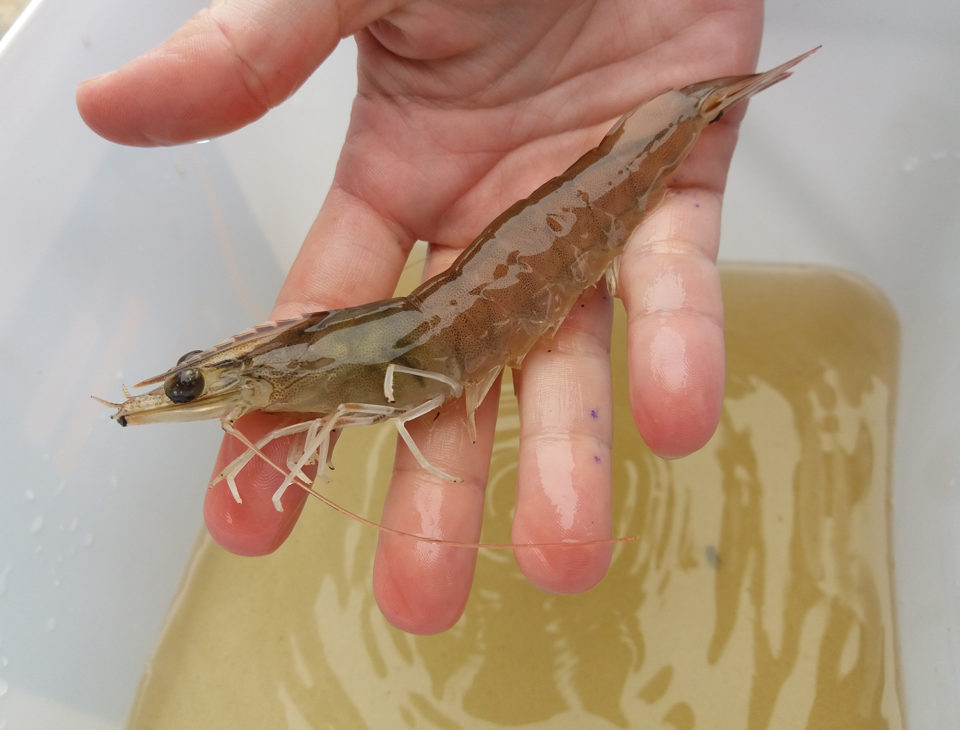
Biofloc technology systems (BFT) are considered one of the most promising technologies in the super-intensive production of aquatic organisms. It has several widely known advantages, including the use of high stocking densities, improved productivity, reduced water consumption (due to little or no water renewal), water reuse, increased biosecurity and the presence of a large microbial community acting as an additional source of food.
Due to the high stocking densities used in BFT and the requirement for high protein concentration in shrimp diets, there is an increase in the amount of ammonium excreted by the animals, which can lead to deterioration of water quality. The metabolization of nitrogen present in water can occur via three pathways: photoautotrophic, where organisms remove the nitrogen of the water turning it into algal biomass; chemoautotrophic, when autotrophic bacteria perform the nitrification process besides incorporating it into its biomass; and the heterotrophic pathway, where bacteria incorporate nitrogen into their biomass in the form of protein.
BFT promotes the development of a community of microorganisms to control nitrogen compounds in water. In most cases, the development of a predominantly heterotrophic bacterial community is promoted by adjusting the carbon-nitrogen ratio of the water and incorporating supplemental organic carbon from carbon-rich organic fertilizers. This incorporation of organic carbon is usually done to adjust the C:N ratio to 15:1, where it has been determined that 15 grams of carbon are needed to convert 1 gram of ammoniacal nitrogen into bacterial biomass.
Some studies suggest that a mixed biofloc system dominated by microalgae and autotrophic bacteria can generate more benefits to the shrimp’s performance, in addition to reducing organic fertilization costs. Other researchers point out that the heterotrophic-chemoautotrophic mixed system is the best alternative, while some promote a completely heterotrophic system.
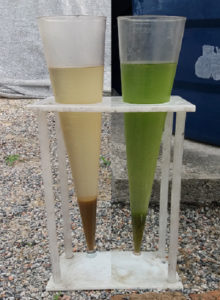
The predominance of the heterotrophic system pathway can generate excess bacterial biomass and cause various problems, such as increasing oxygen demand and carbon dioxide production, leading to water quality alterations, and even affecting the zootechnical performance of shrimp.
Moreover, the chemoautotrophic and photoautotrophic pathways have disadvantages due to being easily removed from the system or creating high fluctuations in water quality parameters. In spite of this, all routes of removal are present in a BFT system and are acting at varying levels and periods, but the autotrophic and heterotrophic pathways seem to have more importance.
Nevertheless, little is known about the formation, composition, development and functioning of bacterial communities in biofloc systems and their concrete connections with the manipulation of the carbon-nitrogen ratio – these studies are essential to characterize the bacterial communities. It is necessary to evaluate the relationship between organic fertilization rates and the development of microbial communities, as well as to characterize them to promote a more efficient use of these communities and reduce the volume of total suspended solids produced.
Fluorescent in situ Hybridization, or FISH
Different techniques are used to describe a bacterial community, such as staining, a method of filtering membrane or culture of bacterial colonies, for example. Although widely used, these techniques may not be very efficient, because they depend on whether the bacteria are cultivable, or on them being non-specific. Molecular techniques, such as electrophoresis, PCR, metagenomics and cytogenetic techniques, have been gaining prominence in recent times due to high performance, precision and speed even though some of them are still very expensive. They make it possible to detail bacterial communities present in the culture water, in cultivated organisms, and even detect the presence of pathogenic or probiotic microorganisms.
FISH (Fluorescent in situ Hybridization) is a molecular technique that uses fluorescent probes attached to oligonucleotides complementary to the ribosomal RNA of bacteria. Fluorescent probes can be designed to be specific and recognize only one species or large bacterial groups. The cells labeled with the probes are differentiated by the use of specific optical filters on the flow cytometer, or epifluorescence or confocal microscopes. In general, the FISH allows direct visualization, identification and computation of specifically labeled bacterial cells with additional advantages of being an independent bacterial culture technique, using negative control to ensure the efficiency of hybridization and providing information about morphology and number of cells in a sample.

C:N manipulation study
A recent study at the Aquaculture Marine Station at the Federal University of Rio Grande (Brazil) showed that it is possible to manipulate the C/N ratio in order to reduce solids production and water use. An experiment with different C:N ratios was carried out, where treatments without supplemental organic fertilization were tested (the entry of organic carbon was done only by feed – C:N = 7.5 / 1) with increasing levels up to C:N = 15:1. Juveniles of Litopenaeus vannamei with initial weight of 1.0 gram were stocked in tanks at the stocking density of 400 per cubic meter.
Water quality parameters such as temperature, pH, dissolved oxygen, ammoniacal nitrogen, alkalinity, nitrate, phosphate, salinity, total suspended solids and turbidity have been monitored. Water renewals were always performed when ammonia levels exceeded 7.0 mg/L and nitrite 20 mg/L (twice the safety level of each in that specific case), or when total suspended solids exceeded 500 mg/L. Water samples were collected to detect the appearance and growth of bacterial populations through FISH technique.
Brandão, Table 1
Parameters | 7.5:1 | 10:1 | 12.5:1 | 15:1 |
---|---|---|---|---|
Initial weight (g) | 1.17±0.50 | 1.17±0.50 | 1.17±0.50 | 1.17±0.50 |
Final weight (g) | 4.62±1.53b | 5.54±1.75a | 5.23±1.81a | 5.45±1.67a |
Initial biomass (g) | 374.40 | 374.40 | 374.40 | 374.40 |
Final biomass (g) | 1283.32 ± 280 | 1618.49 ± 262.83 | 1464.40 ± 124.96 | 1601.52 ± 121.18 |
Final productivity (kg.m³) | 1.60±0.35 | 2.02±0.33 | 1.83±0.16 | 2.00±0.15 |
Weekly growth (g/week) | 0.69±0.6 | 0.87±0.5 | 0.81±0.5 | 0.85±0.5 |
Survival (%) | 86.77±8.03 | 91.35±6.21 | 87.5±8.40 | 91.88±3.48 |
Feed conversion ratio (FCR) | 1.92±0. 47 | 1.50±0.23 | 1.50±0.23 | 1.52±0.11 |
There were no significant differences in water quality parameters, being all within the recommended range for the species. The average concentration of ammonia was the only parameter of water quality affected by the treatments, since higher peaks and means were registered where there was no organic fertilization (Fig. 3). This behavior is due to the smaller amounts of heterotrophic bacteria in that treatment due to the absence of supplemental organic fertilization, as expressed by the FISH results.
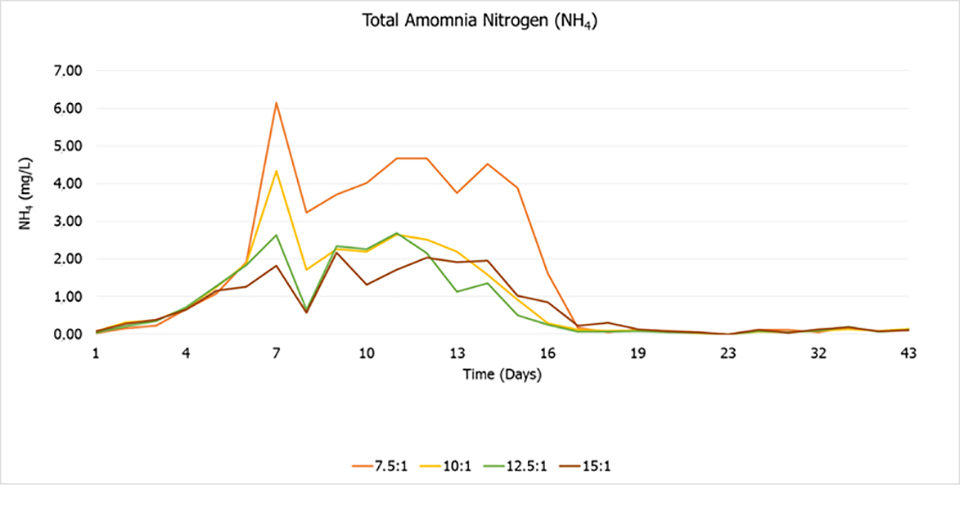
The results also showed that the bacterial community is affected by the C:N ratio, so that the nitrification process was affected by the treatments (Fig. 4), being delayed when the ratio was low (7.5:1) or higher (15:1). Therefore, treatments with intermediate organic fertilization (10:1 and 12.5:1) had the lowest total values for water used and estimated solids removed in the cycle (Fig. 5).

There were also differences in the final weight of shrimp, where the treatment without fertilization obtained the lowest final weight, but no differences were found in the rates of survival, feed conversion and productivity.
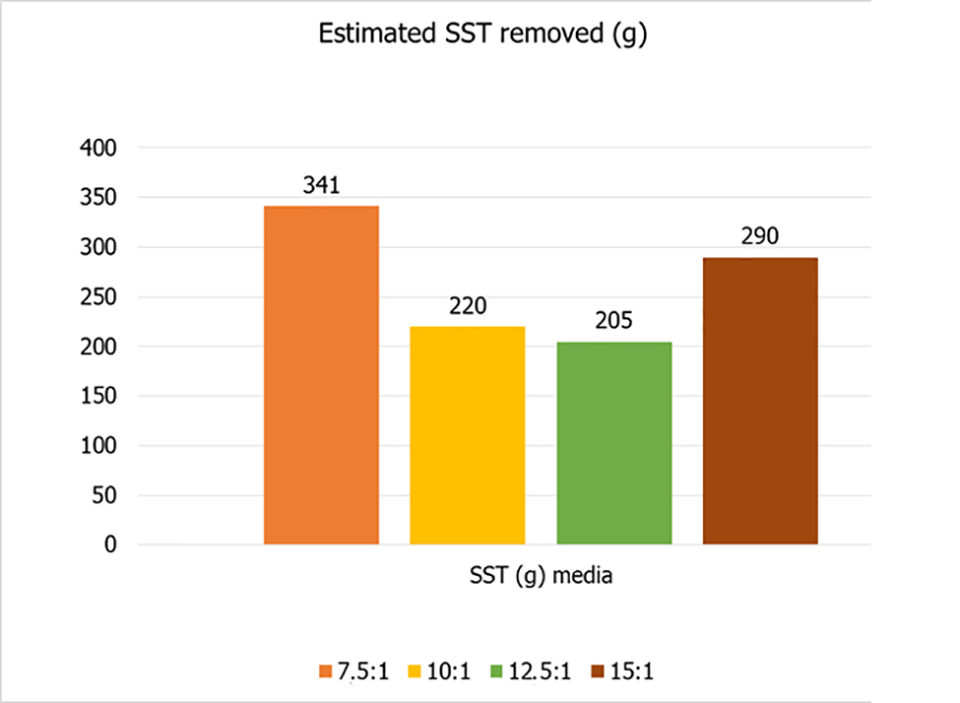
Perspectives
In general, the results showed that it is possible to reduce the carbon-nitrogen ratio in biofloc cultures to reduce the amount of water used and total suspended solids produced, thus generating capital and resource savings. The best C:N ratio depends on the type of desirable biofloc system. The 12.5:1 ratio is recommended when considering the establishment of a mixed heterotrophic-autotrophic system.
Authors
-
Hellyjúnyor Brandão
Laboratório de Cultivo de Camarões
Aquaculture Postgraduate Program
Federal University of Rio Grande – RS, Brazil -
Inácio Alves Neto
Laboratório de Cultivo de Camarões
Aquaculture Postgraduate Program
Federal University of Rio Grande – RS, Brazil -
Dionéia Cesar, Ph.D.
Laboratory of Ecology and Molecular Biology of Microorganisms
Federal University of Juiz de Fora – MG, Brazil -
Carlos Gaona, Ph.D.
Laboratório de Cultivo de Camarões
Aquaculture Postgraduate Program
Federal University of Rio Grande – RS, Brazil -
Dariano Krummenauer, Ph.D.
Laboratório de Cultivo de Camarões
Aquaculture Postgraduate Program
Federal University of Rio Grande – RS, Brazil -
Wilson Wasielesky Jr., Ph.D.
Laboratório de Cultivo de Camarões
Aquaculture Postgraduate Program
Federal University of Rio Grande – RS, Brazil[109,111,99,46,115,117,114,107,105,109,64,119,111,110,97,109]
Tagged With
Related Posts

Aquafeeds
Biofloc and clear-water RAS systems: a comparison
This study compared two types of indoor, shrimp culture systems: clear-water RAS and biofloc systems. Clearwater RAS had the edge in water quality, but shrimp in the biofloc treatment had a higher feed conversion ratio.

Aquafeeds
Biofloc consumption by Pacific white shrimp postlarvae
The stable isotopes technique with δ13C and δ15N can be used to determine the relevance of different food sources to shrimp feeding during the pre-nursery phase of Litopenaeus vannamei culture. During this trial, different types of commercial feed, microalgae, Artemia sp. nauplii and bioflocs were used as food sources.

Intelligence
Biofloc technology production promising in temperate zones
A study was conducted to assess the feasibility to grow Channel catfish (Ictalurus punctatus) in an outdoor biofloc system during winter in a temperate zone. High biomasses of market-size channel catfish were successfully maintained through the winter with high survival and in good condition in both treatments.
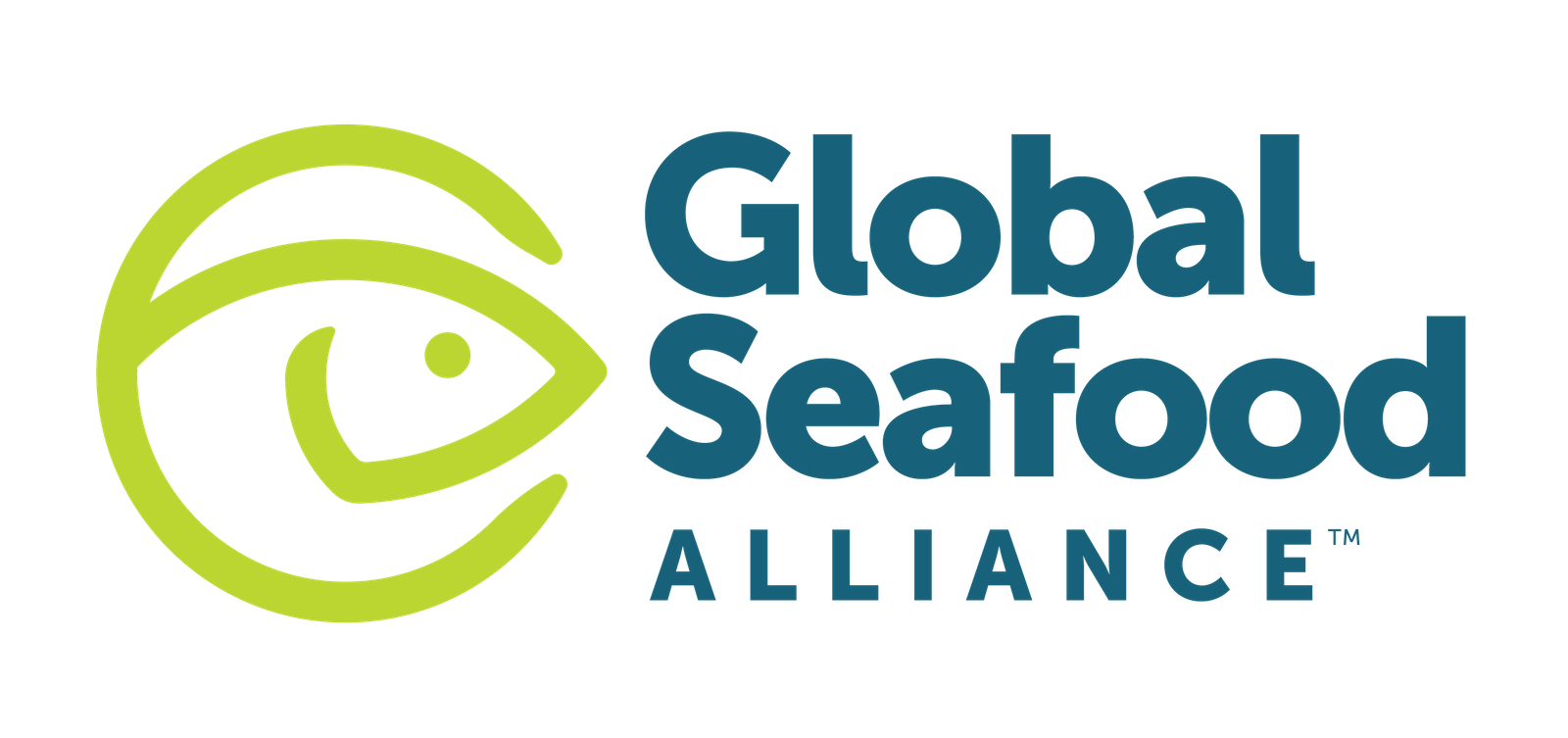
Health & Welfare
Aquamimicry: A revolutionary concept for shrimp farming
Aquamimicry simulates natural, estuarine production conditions by creating zooplankton blooms as supplemental nutrition to the cultured shrimp, and beneficial bacteria to maintain water quality. Better-quality shrimp can be produced at lower cost and in a more sustainable manner.