Use carbohydrates for short-term ammonia-nitrogen control

For numerous reasons, most importantly biosecurity, intensive aquaculture production is moving toward high-density recirculating systems. In these systems, the strategy most commonly used for controlling ammonia-nitrogen and nitrite-nitrogen is to utilize large fixed-film bioreactors that rely on the nitrification of ammonia-nitrogen to nitrate-nitrogen by ammonia-oxidizing bacteria and nitrite-oxidizing bacteria. One of the drawbacks when utilizing bioreactors is the extended time it can take to establish a healthy bacterial biofilm during initial start-up, especially in saltwater systems.
The authors recently experienced a problem often confronted in either new or established facilities – the fish arrived before the biofilters were fully acclimated. In this case, the system had just gone through standard biosecurity wash-down protocols and the moving-bed bioreactor (MBBR) media was as clean as the day it was purchased.
When the live-haul truck with the hybrid striped bass fingerlings arrived, the fish had to go somewhere, so into the tanks they went. Normally, several weeks are required to develop the appropriate biofilm through the addition of ammonia chloride, sodium nitrite and perhaps a commercial starter culture.
C:N ratio manipulation
Over the past several years, the authors have promoted the use of zero-exchange production systems for marine shrimp, where the ammonia build-up is controlled by the manipulation of the carbon-nitrogen ratio (C:N) to promote the growth of heterotrophic bacteria. As a result, the ammonia-nitrogen is removed from the system primarily through assimilation into microbial biomass.
The heterotrophic bacteria impact water quality in several ways:
- The reaction utilizes carbon from carbohydrate as the primary carbon source.
- The pH of the system drops as the alkalinity is reduced.
- Carbon dioxide is produced.
- Oxygen is consumed.
There are several other important impacts due to the heterotrophic bacterial reaction. Paramount is the extremely large amount of bacterial biomass produced and the fast growth rates of the heterotrophic bacteria compared to the autotrophic reaction. This translates into substantial quantities of total suspended solids (TSS) produced, so some form of solids management is required to remove excess TSS production.
Bass production system
At the authors’ bass facility, the production system consisted of a 7.57-cubic-meter round fiberglass grow-out tank with a propeller-washed bead filter for solids capture, a 380-L MBBR with a 50 percent fill ratio, three 2-kW heaters and six 30.5-cm air stones for aeration and carbon dioxide stripping. The system was drained, cleaned, disinfected and refilled with municipal water several days before the bass arrived.
The tank was stocked with 400, 10-gram hybrid striped bass fingerlings fed 200 g of a 45 percent-protein fry diet twice daily. Within several days, the ammonia-nitrogen concentrations had risen to over 3 mg/L, and the only option available appeared to be extensive flushing of the tank with clean freshwater. Although this was a viable option in this case, it usually is not in marine systems due to the high cost of seawater or its lack of availability.
Based on past experiences with zero-exchange marine shrimp systems, it was decided to explore the impacts of adding carbohydrate such as sugar to the system to reduce the ammonia-nitrogen loading on the biofilter.
For numerous reasons, most importantly biosecurity, intensive aquaculture production is moving toward high-density recirculating systems. In these systems, the strategy most commonly used for controlling ammonia-nitrogen and nitrite-nitrogen is to utilize large fixed-film bioreactors that rely on the nitrification of ammonia-nitrogen to nitrate-nitrogen by ammonia-oxidizing bacteria and nitrite-oxidizing bacteria. One of the drawbacks when utilizing bioreactors is the extended time it can take to establish a healthy bacterial biofilm during initial start-up, especially in saltwater systems.
The authors recently experienced a problem often confronted in either new or established facilities – the fish arrived before the biofilters were fully acclimated. In this case, the system had just gone through standard biosecurity wash-down protocols and the moving-bed bioreactor (MBBR) media was as clean as the day it was purchased.
When the live-haul truck with the hybrid striped bass fingerlings arrived, the fish had to go somewhere, so into the tanks they went. Normally, several weeks are required to develop the appropriate biofilm through the addition of ammonia chloride, sodium nitrite and perhaps a commercial starter culture.
C:N ratio manipulation
Over the past several years, the authors have promoted the use of zero-exchange production systems for marine shrimp, where the ammonia build-up is controlled by the manipulation of the carbon:nitrogen (C:N) ratio to promote the growth of heterotrophic bacteria. As a result, the ammonia-nitrogen is removed from the system primarily through assimilation into microbial biomass.
The heterotrophic bacteria impact water quality in several ways:
- The reaction utilizes carbon from carbohydrate as the primary carbon source.
- The pH of the system drops as the alkalinity is reduced.
- Carbon dioxide is produced.
- Oxygen is consumed.
There are several other important impacts due to the heterotrophic bacterial reaction. Paramount is the extremely large amount of bacterial biomass produced and the fast growth rates of the heterotrophic bacteria compared to the autotrophic reaction. This translates into substantial quantities of total suspended solids (TSS) produced, so some form of solids management is required to remove excess TSS production.
Bass production system
At the authors’ bass facility, the production system consisted of a 7.57-cubic-meter round fiberglass grow-out tank with a propeller-washed bead filter for solids capture, a 380-L MBBR with a 50 percent fill ratio, three 2-kW heaters and six 30.5-cm air stones for aeration and carbon dioxide stripping. The system was drained, cleaned, disinfected and refilled with municipal water several days before the bass arrived.
The tank was stocked with 400 10-gram hybrid striped bass fingerlings fed 200 grams of a 45 percent protein fry diet twice daily. Within several days, the ammonia-nitrogen concentrations had risen to over 3 mg/L, and the only option available appeared to be extensive flushing of the tank with clean freshwater. Although this was a viable option in this case, it usually is not in marine systems due to the high cost of seawater or its lack of availability.
Based on past experiences with zero-exchange marine shrimp systems, it was decided to explore the impacts of adding carbohydrate such as sugar to the system to reduce the ammonia-nitrogen loading on the biofilter. Four treatments were tried over several days: 50, 100, 150 and finally 200 percent of the daily feed rate was added as sugar.
Figure 1 shows the impacts of the sugar on the total ammonia-nitrogen levels as measured with a spectrophotometer following standard methods. The data was normalized to the 50 percent feed rate, which corresponded to the approximate amount of additional organic carbon necessary to completely satisfy the carbon demand for the ammonia-nitrogen generated by 200 grams of feed (8.28 grams of nitrogen). Since about 6.07 grams of carbon are needed per gram of nitrogen, approximately 50 grams of organic carbon were needed from the feed or added as carbohydrate.
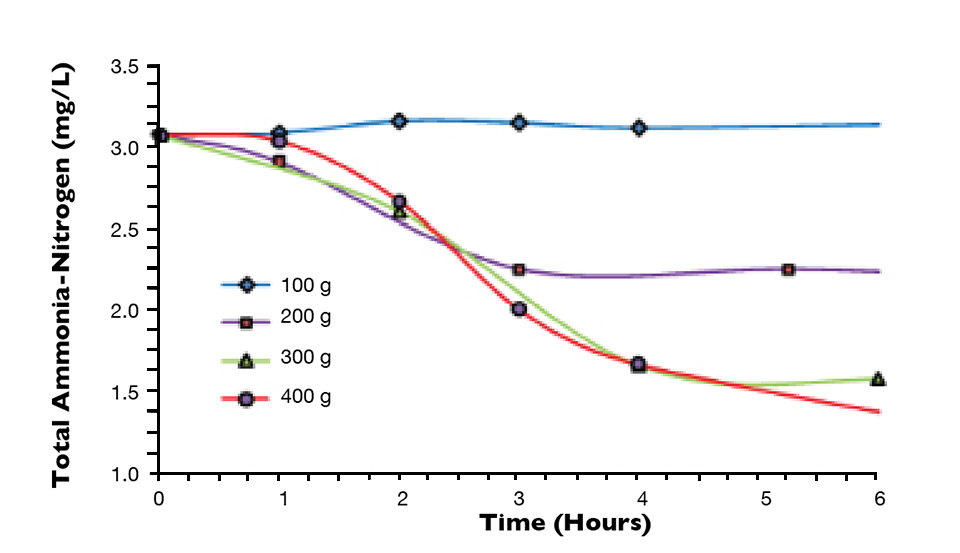
The organic carbon available from feed is difficult to quantify and depends on numerous conditions, including the composition of the feed, the assimilation rate of the culture species, the effectiveness of the solids capture device and the use of foam fractionators or ozone.
It is readily apparent in Fig. 1 that for this case, the addition of 50 percent of the feed as sugar provided sufficient organic carbon to completely neutralize the ammonia-nitrogen produced by assimilating it into heterotrophic bacteria biomass as previously described. Not only was the ammonia-nitrogen removed from the system as TSS, but there was no production of either nitrite-nitrogen or nitrate-nitrogen.
Impacts on water quality
Three important impacts on overall water quality with this technique are the production of TSS, production of carbon dioxide and consumption of oxygen. Using heterotrophic bacteria to remove the ammonia-nitrogen generated produces significant quantities of TSS and requires an efficient solids-capture system to maintain system water quality. Luckily, during start-up, the total quantity of feed is at its lowest value, so this should not be a major problem.
The heterotrophic bacteria also generate a significant quantity of carbon dioxide, as seen in Fig. 2, which shows the pH of the system dropped substantially over the first three or four hours. In this case, there was sufficient aeration to off gas the carbon dioxide.

Finally, sugar could be characterized as a “hot source” of organic carbon, in that the effects were apparent within a very short time. Dissolved oxygen can quickly drop due to the high demand of the fast-growing bacteria, so adequate aeration is essential. It might be possible to use other carbohydrate sources that release organic carbon more slowly.
The estimated total ammonia-nitrogen removed at each sugar-dosing level was plotted to estimate the removal rate of nitrogen as a function of the sugar dose (Fig. 3). The data were amazingly consistent with a slope through zero of 16.4 grams sugar per gram of nitrogen removed.

Perspectives
Although not yet established as a long-term production strategy, the use of carbohydrates for the short-term control of ammonia-nitrogen worked quite well in this study. In addition, the media in both the bead filter and the MBBR was coated with a thin layer of heterotrophic bacteria that made the media “sticky.”
In the MBBR, this provided a more congenial surface on which other bacteria – hopefully the nitrifying bacteria initially intended to provide ammonia and nitrite control – could colonize. In the bead filter, this served to increase fine solids removal efficiency.
After only five days of this study, it was decided to add a control with no sugar to follow proper scientific protocols, but the biofilter had already become activated and the ammonia-nitrogen levels in the tank were below 0.3 mg/L.
(Editor’s Note: This article was originally published in the July/August 2009 print edition of the Global Aquaculture Advocate.)
Now that you've finished reading the article ...
… we hope you’ll consider supporting our mission to document the evolution of the global aquaculture industry and share our vast network of contributors’ expansive knowledge every week.
By becoming a Global Seafood Alliance member, you’re ensuring that all of the pre-competitive work we do through member benefits, resources and events can continue. Individual membership costs just $50 a year. GSA individual and corporate members receive complimentary access to a series of GOAL virtual events beginning in April. Join now.
Not a GSA member? Join us.
Authors
-
James M. Ebeling, Ph.D.
Aquaculture Systems Technologies, LLC
108 Industrial Avenue
New Orleans, Louisiana 70121 USA[109,111,99,46,115,114,101,116,108,105,102,100,97,101,98,64,115,101,109,97,106]
-
Dr. Michael B. Timmons, P.E.
Biological and Environmental
Engineering Department
Cornell University
Ithaca, New York, USA
Tagged With
Related Posts

Health & Welfare
Biofilter inoculation in recirculating aquaculture systems
Biological filters are essential parts of recirculating aquaculture systems that transform toxic fish compounds such as ammonium and nitrite into less-harmful nitrate. The authors tested the convenience and efficiency of three methods for the initial inoculation of aerobic biofilters.

Innovation & Investment
Estimating biofilter size for RAS systems
Controlling total ammonia-nitrogen (TAN) concentrations is the primary concern when sizing a biofilter for use in a recirculating aquaculture system. Sizing decisions are best based on previous experience with a given biofilter media in a specific biofilter configuration.

Responsibility
A look at unit processes in RAS systems
The ability to maintain adequate oxygen levels can be a limiting factor in carrying capacities for RAS. The amount of oxygen required is largely dictated by the feed rate and length of time waste solids remain within the systems.

Innovation & Investment
A review of unit processes in RAS systems
Since un-ionized ammonia-nitrogen and nitrite-nitrogen are toxic to most finfish, controlling their concentrations in culture tanks is a primary objective in the design of recirculating aquaculture systems.