Pathways for nitrogen removal differ in terms of substrate utilization, bacterial biomass generated and byproducts produced
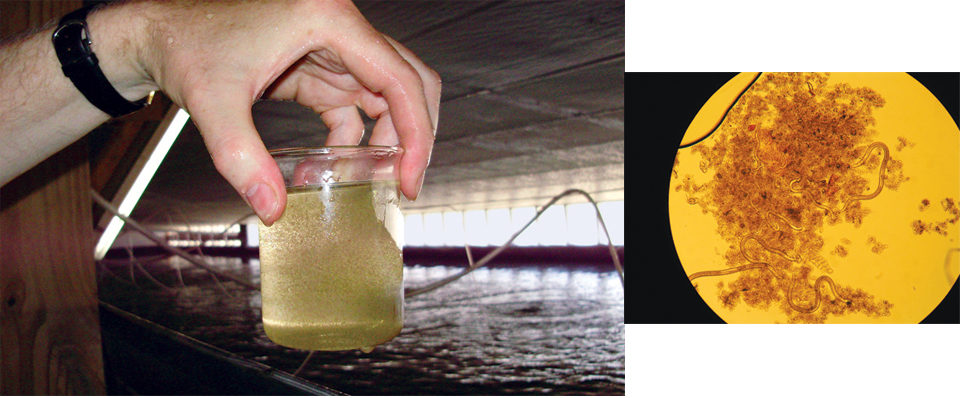
In intensive aquaculture systems, ammonia-nitrogen build-up from the metabolism of feed is usually the next limiting factor, after dissolved oxygen, for increasing production levels. The three pathways for the removal of ammonia-nitrogen in traditional systems are consumption by photoautotrophic algae, autotrophic bacterial conversion from ammonia-nitrogen to nitrate-nitrogen and heterotrophic bacterial conversion from ammonia-nitrogen directly to microbial biomass, a more recent method.
Traditionally, pond aquaculture used photoautotrophic algae-based greenwater systems to control inorganic nitrogen utilizing carbon dioxide as a carbon source. In intensive recirculating production, large fixed-cell bioreactors that rely on the nitrification of ammonia-nitrogen to nitrate-nitrogen by autotrophic bacteria utilizing inorganic carbon are routinely used. In these systems, the accumulation of organic carbon required for the heterotrophic bacteria is intentionally minimized through water exchange and the rapid removal of solids.
In contrast, the inorganic nitrogen build-up in microbial-based production systems can be controlled by manipulation of the organic carbon:nitrogen (C:N) ratio to promote the growth of heterotrophic bacteria. The heterotrophic bacteria using the organic carbon assimilate ammonia-nitrogen directly from the water column, producing cellular protein that can also act as a supplemental food source for marine shrimp and other species.
Roles of organic, inorganic carbon
In the authors’ experience, there is often confusion as to the roles played by organic and inorganic carbon in selecting ammonia removal pathways. The pathways are to some degree determined by the form of carbon available: inorganic carbon (alkalinity or carbon dioxide) or organic carbon (uneaten feed and fecal matter or supplemental carbohydrates). Thus, for a system where all of the solids containing organic carbon are rapidly removed, the system would be primarily autotrophic, utilizing inorganic carbon from the alkalinity as its carbon source.
For a microbial-based system, the solids remain and all of the carbon and nitrogen from the feed and fecal matter is available for heterotrophic bacterial production. In this case, because there is usually insufficient organic carbon for the bacteria to metabolize all the available ammonia-nitrogen, some limited autotrophic conversion occurs, utilizing inorganic carbon from alkalinity. If sufficient supplemental organic carbon is added – as carbohydrates, for example – the nitrogen is converted to bacterial biomass via heterotrophic bacteria.
These two pathways for nitrogen removal are very different in terms of substrate utilization, bacterial biomass generated and by-products produced.
Autotrophic systems
For a pure autotrophic nitrification process, the two forms of nitrifying bacteria utilize the inorganic form of carbon (alkalinity) for cellular growth, first converting ammonia-nitrogen into nitrite-nitrogen and then nitrate-nitrogen. These autotrophic bacteria grow very slowly and generate only a small amount of volatile suspended solids (VSS), approximately 10 grams VSS/kg feed at 35 percent protein.
Almost 355 grams of alkalinity as calcium carbonate/kg of feed is consumed by the autotrophic bacteria, but only about 6 percent is actually used for biomass production. The rest is released as carbon dioxide. The C:N ratio for optimal conversion by autotrophic systems can be stoichiometrically determined as about 1.69 grams of inorganic carbon per gram nitrogen, compared to the microbial biomass C:N ratio of 4.28 grams of organic carbon per gram nitrogen.
Microbial-based heterotrophic systems
Consider a microbial-based system where all of the solids remain in the production tank, and all of the organic carbon and nitrogen from the uneaten feed and fecal matter is available for heterotrophic bacterial production. Since the energetics for heterotrophic bacteria are more favorable than for autotrophic bacteria, it can be assumed that the heterotrophic bacteria will first consume the available nitrogen using the readily available, labile carbon from the uneaten feed and fecal matter. The remaining nitrogen will then be assimilated by the autotrophic bacteria using inorganic carbon.
One problem, though, is estimating the available organic carbon from the uneaten feed and fecal matter, due to the wide variations in feed formulations, species assimilation, nutrient leaching from the feed particles and numerous other difficulties. Thus, as an approximation, literature data was used to estimate the biochemical oxygen demand (BOD) per kilogram of feed and yield fraction of VSS per kilogram BOD. The stoichiometric relationships were then used to estimate the organic carbon and nitrogen sequestered in the heterotrophic microbial biomass. The remaining nitrogen is converted to nitrate by the autotrophic bacteria.
Two forms of carbon are consumed along this pathway: 109.0 g of organic carbon by the heterotrophic bacteria and 15.4 grams of inorganic carbon by the autotrophic bacteria per kilogram of feed at 35 percent protein. The resulting C:N ratio based on the labile organic carbon is 2.16, and based on total carbon, 8 to 10. Although the exact value depends on the protein content, for 35 percent-protein feed, 35.6 percent of the nitrogen is removed by the heterotrophic pathway and 64.4 percent by the autotrophic pathway.
The percentage of the nitrogen assimilated by the heterotrophic bacteria compared to the autotrophic bacteria is a function of the available organic carbon and the nitrogen in the feed (Fig. 1). At a very low protein content, the feed contains sufficient labile organic carbon to completely assimilate all of the nitrogen.
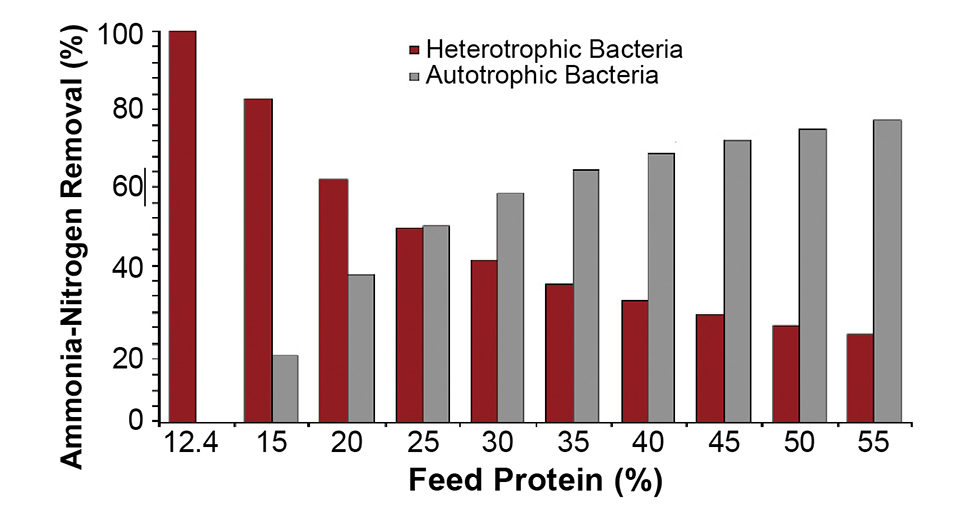
This explains the success of microbial floc-based systems for marine shrimp production, where low-protein feed is initially used to stimulate the production of heterotrophic bacteria. In addition, compared to pure autotrophic systems, significant quantities of bacterial biomass are generated by the heterotrophic bacteria – 150.5 grams VSS/kg feed at 35 percent protein.
Consider a zero-exchange system where carbon is added to make up the difference between the available organic carbon from 1 kg of 35 percent-protein feed (109 grams) and the requirements of the heterotrophic bacteria are approximately 306 grams of organic carbon. The remaining 197 grams of organic carbon must be compensated for with, for example, 492 grams of carbohydrate or 49 percent by weight of the feed.
Thus, a total of 306 grams of organic carbon would be required to convert heterotrophically 50.4 grams of nitrogen. This yields a C:N ratio of 6.07. Fig. 2 graphically shows the supplemental carbohydrate requirement as a percentage of feed rates required for complete heterotrophic metabolism of ammonia-nitrogen to microbial biomass.

Finally, please note that a pure heterotrophic system generates approximately 406 grams VSS/kg feed at 35 percent protein, more than 40 times that of a pure autotrophic system. This microbial biomass can be a supplemental food source or must be removed from the production system.
Outlook
The two pathways for nitrogen removal are very different in terms of substrate utilization, bacterial biomass generated and byproducts produced. Using the stoichiometric relationships for autotrophic and heterotrophic bacteria, it is possible to model the two pathways for nitrogen removal typically found in microbial-based systems. The difficulty in practical application is that both can be active to some degree, depending upon the availability of inorganic and organic carbon.
Results of this model and lab research suggested that not all of the organic carbon in feed is readily available to the heterotrophic bacteria. For example, only 109 grams per kg of labile organic carbon is contained in 35 percent-protein feed compared to a proximate analysis of 350-400 grams carbon per kg feed. The ability to control the type of carbon and C:N ratio by feed formulation, solids removal or addition of organic carbon allows aquaculture producers to manage which type of pathway becomes dominant in their systems.
(Editor’s Note: This article was originally published in the July/August 2008 print edition of the Global Aquaculture Advocate.)
Now that you've finished reading the article ...
… we hope you’ll consider supporting our mission to document the evolution of the global aquaculture industry and share our vast network of contributors’ expansive knowledge every week.
By becoming a Global Seafood Alliance member, you’re ensuring that all of the pre-competitive work we do through member benefits, resources and events can continue. Individual membership costs just $50 a year. GSA individual and corporate members receive complimentary access to a series of GOAL virtual events beginning in April. Join now.
Not a GSA member? Join us.
Authors
-
James M. Ebeling, Ph.D.
Aquaculture Systems Technologies, LLC
108 Industrial Avenue
New Orleans, Louisiana 70121 USA[109,111,99,46,115,114,101,116,108,105,102,100,97,101,98,64,115,101,109,97,106]
-
Michael B. Timmons, Ph.D.
Department of Biological and Environmental Engineering
Cornell University
Ithaca, New York, USA
Related Posts

Responsibility
Ammonia nitrogen dynamics in aquaculture
The major sources of ammonia in aquaculture ponds are fertilizers and feeds, and problems with high ammonia are most common in feed-based aquaculture.

Responsibility
A look at unit processes in RAS systems
The ability to maintain adequate oxygen levels can be a limiting factor in carrying capacities for RAS. The amount of oxygen required is largely dictated by the feed rate and length of time waste solids remain within the systems.

Responsibility
A look at various intensive shrimp farming systems in Asia
The impact of diseases led some Asian shrimp farming countries to develop biofloc and recirculation aquaculture system (RAS) production technologies. Treating incoming water for culture operations and wastewater treatment are biosecurity measures for disease prevention and control.

Innovation & Investment
A review of unit processes in RAS systems
Since un-ionized ammonia-nitrogen and nitrite-nitrogen are toxic to most finfish, controlling their concentrations in culture tanks is a primary objective in the design of recirculating aquaculture systems.