Establishment of heterotrophic bacterial communities
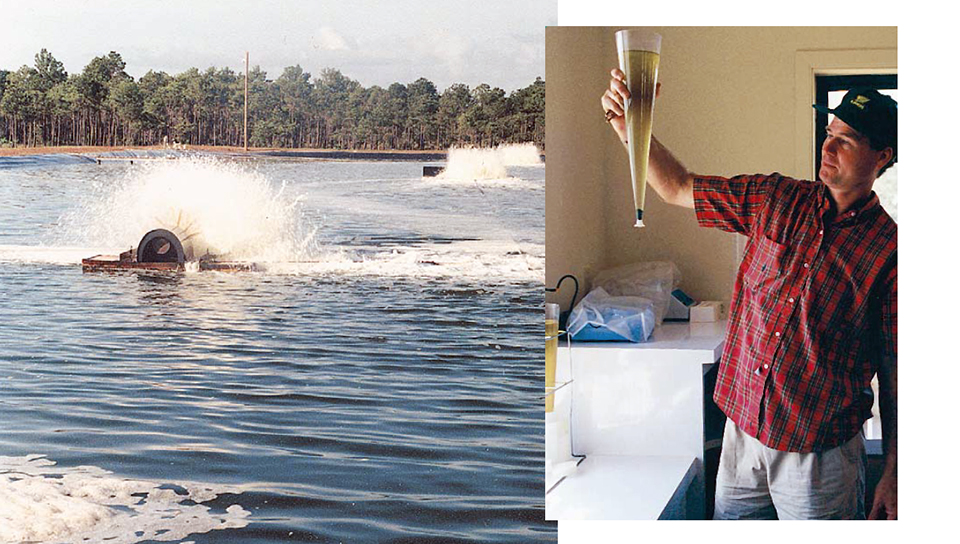
Belize Aquaculture, Ltd. successfully uses a zero-water exchange production strategy to reduce effluents, increase biosecurity, and generate high yields. Shrimp grown under heterotrophic conditions here have an excellent flavor and are well received in the marketplace. The discussion begun in the December 2000 issue of the Advocate on development of the heterotrophic systems in shrimp ponds at Belize Aquaculture is concluded here.
Organic material and C:N ratio

In addition to high stocking rates and adequate pond aeration, the final factor that must be considered in the development of a heterotrophic system is the amount of organic material being fed to a pond, and the carbon per nitrogen balance of that material. Bacteria feed on organic material, and in order to establish a healthy bacterial population, there must be sufficient organics in the water. Equally important is a balance of other nutrients, such as nitrogen, required by bacteria for growth.
In order to transition from an autrophic to a heterotrophic community, it is necessary to add significantly higher amounts of organic material – with a balanced carbon per nitrogen ration – than the shrimp will consume. Initially, be fore we understood our system, we added feeds and organic material with an average carbon per nitrogen ratio of 11:1 (protein level of 30 percent) at Belize Aquaculture.
This resulted in a system that was out of balance, having too much nitrogen for the amount of carbon present. Pond water and bottoms became fouled, and sulfides were often detected. Raising the carbon per nitrogen ratio to 16:1 (protein level of 22 percent) resulted in a very healthy heterotrophic community that appears to be more in balance.
A grain-based pellet with a high carbon per nitrogen ratio of 20:1 (protein level of 18 percent) is used to counterbalance the lower carbon per nitrogen ratios of the shrimp diets being fed. These pellets are added at a rate of 250 kilograms per hectare several days before stocking, and at a rate of 220 percent of biomass for the first week after stocking (representing 90 percent of the organic input). This overfeeding continues until the shrimp grow to 3 grams, at which time feed rates are determined by consumption patterns established by monitoring feed trays.
Molasses boosts initial growth
Recently, we found the use of molasses in ponds can speed up the transition between the autotrophic and heterotrophic states. Applying 50 liters per hectare of sugar cane molasses twice a week to recycled water (nitrogen level of 3 ppm) lead to the development of bacterial flocs within the second week of culture.
Initial shrimp growth rate increases in these ponds were significant, reflecting juveniles that weighed 2.5 grams after four weeks of grow-out. After the bacterial flocs are established, however, there were no obvious advantages to continuing the addition of molasses.
Types of bacterial vommunities
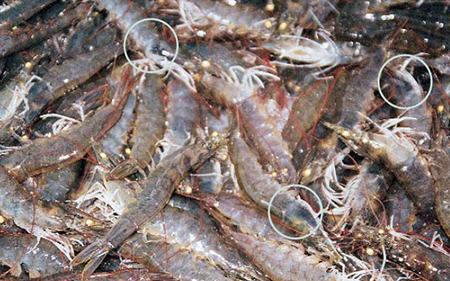
Not all heterotrophic communities are the same. Belize Aquaculture has identified three distinct types of bacterial communities: brown flocs, black flocs and green flocs. Good shrimp crops have been obtained with all three community types, but we prefer the brown flocs, as shrimp from these ponds have shells with a shiny, healthy appearance and negligible scaring.
Shrimp from ponds with black flocs always develop black gills. It has been determined that black flocs have much higher iron levels than do brown flocs (Rod McNeil, personal communication). I believe black gills are the result of colonization in the gill tissue of shrimp by the same bacteria that make up the black flocs. The bacteria deposit iron on the shrimps’ gill lamellae.
Black flocs developed approximately 40 percent of the time during Belize Aquaculture’s first two years of operation (1998-1999), but they occurred in only 5 percent of the ponds in 2000. Brown flocs now predominate, and black flocs developed in only 5 percent of the ponds in 2000. By pumping water from a pond with brown flocs into newly filled ponds, some control seems to be possible in selecting the type of bacteria community that will dominate the pond.
An interesting question is why bacteria so completely dominate the ecology of the system. The pond water always has sufficient nutrients to grow phytoplankton, but about the only phytoplankton observable are a few phytoflagellates, which are known to be capable of both autotrophy and heterotrophy. There is sufficient sunlight, and ponds would typically have a transparency of 30 cm using a Secchi disk. A possible explanation is the production of lysozymes by the bacteria, which digest phytoplankton cells. This would also explain why the system has such a large capacity to handle feeding rates as high as 500 to 600 kilograms per hectare per day.
Water chemistry dynamics

Plastic-lined ponds that do not exchange water develop some interesting water chemistry dynamics. After initial stocking, oxygen levels are typical of semi-intensive nonaerated ponds. As the shrimp biomass increases, and the system converts to heterotrophy, the diurnal difference in oxygen levels becomes less pronounced (Fig. 1).
Instead of oxygen being produced by photosynthesis, more carbon dioxide is produced by both bacterial and shrimp respiration. Free carbon dioxide levels can rise to 15 ppm at the end of a cycle, and it may be carbon dioxide that eventually becomes the factor that limits shrimp growth.
Increases in carbon dioxide levels also result in a lowering of pond water pH to a range of 6.8 to 7.3. The Litopenaeus vannamei cultured at Belize Aquaculture do not seem to react adversely to this pH range. It is probable the lowered pH is beneficial, in that it reduces the level of toxic unionized ammonia.
Ammonia-nitrogen levels increase to 8 to 12 ppm before starting to decline around weeks six to eight. As would be expected, nitrite and nitrate levels begin to increase. Ammonia-nitrogen levels generally decline to less than 3 ppm after week eight of the cycle, but nitrite-nitrogen levels generally do not decline and usually range from 3 to 8 ppm. It appears the system is still limited by carbon relative to nitrogen. The addition of molasses, which further enriches the carbon relative to nitrogen, results in a further decline in both ammonia and nitrite levels, supporting the idea that the system is carbon-limited.
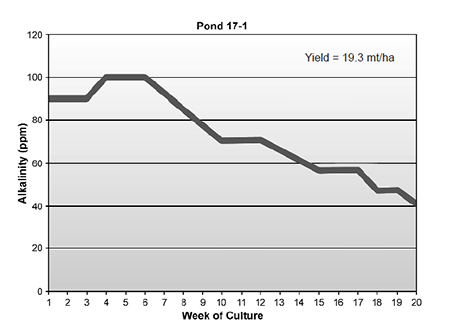
Alkalinity levels (Fig. 2) can decline to less than 20 ppm if there is no supplementation of liming or carbonate materials. It is assumed the reduction in alkalinity is the result of nitrification processes that are taking place within the system.
Total phosphorus levels (Fig. 3) increase linearly with time, and can reach levels higher than 25 ppm as phosphorous. When phytoplankton dominate the culture system, orthophosphate represents less than 20 percent of the total phosphorus. When the system becomes heterotrophic, orthophosphate can represent 80 percent of the total phosphorus. It appears the bacteria do not store phosphorus, as many algae and phytoplankton do.
Because the ponds are lined with high-density polyethylene plastic liners, and the water does not contact bottom clay or other ionic materials that can result in the absorption and removal of phosphorus from the water, phosphorus levels build up with time. However, so far no negative impacts on shrimp growth or health have been attributed to the high phosphorus levels.
Because there is no water exchange, the idea that pond water must have the appearance of thick “pea soup” was popularized. After the heterotrophic state and flocs are established, however, typical Secchi transparency is between 25 and 35 cm.
Management
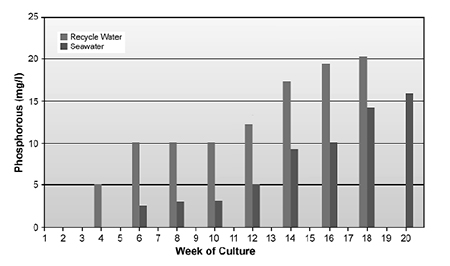
Management of intensive systems using zero water exchange can be easier than management of well-run semiintensive farms. Heterotrophic systems are forgiving when it comes to feeding, and do not require constant monitoring of environmental parameters, or nutrient levels so that fertilization rates can be calculated daily. Heterotrophic systems also do not have a “Ph.D. requirement” to run successfully. Belize Aquaculture’s ponds are managed by a group of dedicated technicians that have high school equivalency only.
Although heterotrophic systems are forgiving in the area of pond management, there is a tradeoff in the area of machine maintenance. A dedicated team is needed to maintain aerators, generators and electrical systems.
Useful chemicals
In the development of the zero-water-exchange culture system, several chemicals have proven useful, including lime, silicate cake, and a product called Aquaculture Pond Stabilizer™ (PVS Technologies, Detroit, Michigan, USA). Hydrated lime or the equivalent is added at a rate of 100 kilograms per hectare every 14 days, starting at week seven in the cycle. This dosage seems to maintain pond alkalinity in the range of 75 to 100 ppm.
Silicate cake, a byproduct of the clay mining industry, is composed of 25 to 45 percent zeolite, 15 to 30 percent kaolin, and 5 to 15 percent sodium silicate. Although this product is not essential, we believe the small particles of the material act as an agent to initiate nucleation of the bacteria into flocs. Silicate cake is added during pond preparation at a rate of 250 kilograms per hectare, and supplemented at 100 kilograms per hectare every four weeks. There are certainly other materials that can be used to facilitate the nucleation of the bacteria into flocs.
Aquaculture Pond Stabilizer is a product that has been effective in neutralizing sulfides that build up in areas of sludge accumulation. Application is made with aerators on, so the material settles into areas where sludge is accumulating. There the material binds and precipitates the sulfide in a non-toxic form. Initial trials with the product have resulted in positive production trends.
Conclusion
Heterotrophic bacterial communities facilitate the production of high yields (~15 metric tons per hectare per cycle) in ponds with zero water exchange. Factors that have helped improve the efficiency of this system include:
- seeding of newly filled ponds with brown floc
- early addition of molasses to advance floc development
- use of feed with high C:N to avoid nitrogen accumulation
- use of lime to maintain alkalinity
- use of silicate to nucleate flocs
- use of Aquaculture Pond Stabilizer to neutralize sulfides.
(Editor’s Note: This article was originally published in the February 2001 print edition of the Global Aquaculture Advocate.)
Now that you've finished reading the article ...
… we hope you’ll consider supporting our mission to document the evolution of the global aquaculture industry and share our vast network of contributors’ expansive knowledge every week.
By becoming a Global Seafood Alliance member, you’re ensuring that all of the pre-competitive work we do through member benefits, resources and events can continue. Individual membership costs just $50 a year. GSA individual and corporate members receive complimentary access to a series of GOAL virtual events beginning in April. Join now.
Not a GSA member? Join us.
Author
-
Robins P. McIntosh
Belize Aquaculture, Ltd.
Belize City, Belize, Central America[116,101,110,46,108,116,98,64,97,117,113,97,101,122,105,108,101,98]
Tagged With
Related Posts

Intelligence
Changing paradigms in shrimp farming, part 5
A zero-water-exchange strategy to produce shrimp reduces the effluents and sediments that would normally be released to the environment by a typical intensive shrimp farm.

Intelligence
Changing paradigms in shrimp farming, part 4
In high-density systems with zero water exchange, pond ecology shifts from an autotrophic, phytoplankton-based microbial community to a heterotrophic, bacterial-based community.

Intelligence
Changing paradigms in shrimp farming, part 3
Square-shaped and deep ponds may explain partially why Belize Aquaculture is able to produce a large amount of shrimp on a unit area basis.

Intelligence
Changing paradigms in shrimp farming, part 1
Conventional wisdom for farming white shrimp involves semi-intensive culture in large ponds, routine water exchange and reliance on wild broodstock.