Understanding, combining LC-PUFA sparing for fish oil replacement
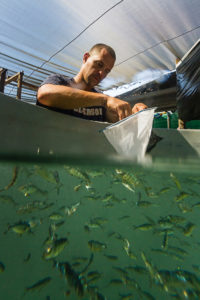
The California yellowtail (Seriola dorsalis), like many other marine carnivorous fish, has dietary requirements for certain long-chain polyunsaturated fatty acids (LC-PUFAs). More precisely, its feeds must include adequate amounts of arachidonic acid (ARA) and docosahexaenoic acid (DHA). And also including eicosapentaenoic acid (EPA) may also be important for optimizing its growth performance.
Consequently, directly replacing LC-PUFA-rich fish oil with other, less costly alternative lipids typically devoid of these nutrients has proven difficult for Seriola spp. Similar information has also been reported for other species with similar essential fatty acid requirements. Adjusting alternative lipid-based feeds with other sources of DHA, EPA and ARA can reestablish performance, but these specialty supplements are costly and reduce the savings resulting through fish oil sparing.
It may be possible to meet the fatty acid requirements of fish like California yellowtail with reduced amounts of costly individual LC-PUFA concentrates if they are fed alternative lipids with little-to-no C18 PUFA content, but results are mixed regarding the relative strength of LC-PUFA sparing associated with SFAs vs. MUFAs. In most, but not all cases, SFA-rich lipids induce a stronger LC-PUFA sparing effect than MUFA-rich lipids, but there has been little work done to assess how much SFA content is enough to induce the sparing effect, whether saturates and unsaturates interact synergistically or antagonistically when both are present in the diet, etc.
In this study, summarized here from the original publication (https://doi.org/10.1016/j.aquaculture.2018.04.040), we compared production performance and tissue fatty acid composition of California yellowtail fed diets with fish oil or soybean oil (rich in C18 PUFAs), partially hydrogenated soybean oil (rich in MUFAs), fully hydrogenated soybean oil (rich in SFAs), or blends of these soybean oils supplemented to meet requirements for LC-PUFAs previously determined.

Study setup
Juvenile California yellowtail (initial individual weight=6.2 ± 0.0 grams, mean ± SEM) were randomly stocked in a semi-closed recirculating aquaculture system equipped with supplemental aeration, mechanical and biological filters at the Hubbs-Sea World Research Institute (HSWRI) facility. Twenty-four, 300-L tanks were stocked with 15 fish/tank, and dietary treatments were randomly assigned to quadruplicate tanks.
The experiment lasted for 10 weeks. Fish were fed continuously with belt feeders, at a ration of approximately 7 percent of body weight/day (equivalent to apparent satiation) equally divided into six feeding events/day. Tanks were monitored regularly for uneaten feed and none was observed during the daily siphoning events to remove solids. All animal husbandry and sampling protocols, including those described below, were in keeping with the HSWRI standards and guidelines for humane animal care and use in research.
Dietary treatments were formulated to contain 473.7 grams per kg of menhaden fish meal and 240 grams per kg of soy protein concentrate. Experimental diets varied only in lipid source and fatty acid composition (e.g. fatty acid groupings such as SFAs, MUFAs and C18 PUFAs) The control diet (“FISH”) was based on previous tested formulations and contained menhaden fish oil as the only supplemental lipid source.
The experimental dietary treatments contained various types of soy-derived lipids as needed to achieve distinct fatty acid profiles favoring different fatty acid groupings, including fully hydrogenated SFA-rich soybean oil (“SFA SOY”diet); partially hydrogenated MUFA-rich soybean oil (“MUFA SOY” diet); standard C18 PUFA-rich soybean oil (“C18 PUFA SOY”diet); a blend of fully and partially hydrogenated soybean oils providing similar levels of SFAs and MUFAs, but low C18 PUFA content (“LOW PUFA BLEND” diet); and another blend containing all soybean oil types providing an approximately equal balance of SFAs, MUFAs, and C18 PUFAs (“BALANCED SOY BLEND”).
To ensure the LC-PUFA requirements of juvenile California yellowtail were met, the soybean oil-based experimental diets were supplemented with LC-PUFA concentrates rich in EPA, DHA and ARA to achieve the necessary levels of these fatty acids; because of the EPA, DHA, and ARA content of menhaden fish oil, the FISH diet did not require supplementation.
The experimental diets used in the study were prepared at the Center for Fisheries, Aquaculture and Aquatic Sciences (CFAAS; Carbondale, Ill., USA) according to standard in-house feed manufacturing procedures. After collecting sub-samples of each diet for compositional analysis diets were sent to the Hubbs-Sea World Research Institute (HSWRI; Carlsbad, Calif., USA) facilities for the feeding trial where they were kept frozen prior to use (minus-20 degrees-C).
For detailed descriptions of the procedures for experimental design and feeding trial; experimental feed preparation and analyses; production performance, sample collection and analyses; tissue fatty acid analyses and coefficient of distance (Djh) values; statistical analyses; and references, please contact the first author.
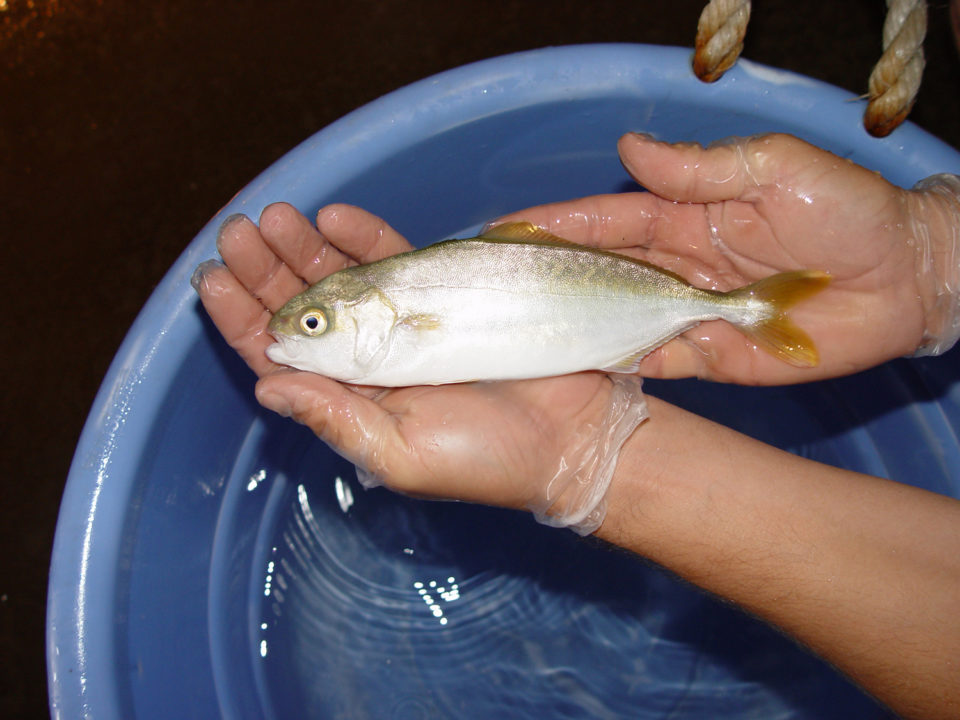
Results and discussion
Overall results show that fish survival during the trial was 100 percent, and that production performance was not affected by dietary lipid source or fatty acid composition (Table 1). Fish growth was relatively fast, with weight gain averaging 1442 ± 20 percent (grand mean ± SEM) and SGR averaging 3.91 ± 0.02 percent body weight/day across all treatment groups. Feed intake (6.59to 6.82 percent body weight/day) and FCR (1.25to 1.33) were also unaffected by dietary treatment. And the organosomatic indices were not significantly different, and differed little among the treatment groups.
Rombenso, CYT, Table 1
Production variable | FISH | SFA SOY | MUFA SOY | C18 PUFA SOY | LOW PUFA BLEND | BALANCED SOY BLEND | PSE | P value |
---|---|---|---|---|---|---|---|---|
Initial weight (g) | 6.2 | 6.2 | 6.22 | 6.3 | 6.2 | 6.3 | 0.1 | 0.566 |
Final weight (g) | 96.2 | 95.5 | 97.5 | 91.5 | 99.2 | 98.4 | 3.2 | 0.258 |
Weight gain (%) | 1454 | 1431 | 1470 | 1351 | 1488 | 1457 | 50 | 0.156 |
FCR | 1.3 | 1.26 | 1.26 | 1.33 | 1.25 | 1.29 | 0.03 | 0.356 |
SGR (% body weight/day) | 3.92 | 3.90 | 3.93 | 3.82 | 3.95 | 3.93 | 0.05 | 0.127 |
Feed intake (% body weight/day) | 6.82 | 6.59 | 6.69 | 6.71 | 6.68 | 6.80 | 0.11 | 0.316 |
HIS | 10 | 0.9 | 1.0 | 1.0 | 0.9 | 1.0 | 0.1 | 0.925 |
VSI | 7.2 | 7.1 | 7.0 | 7.0 | 7.1 | 7.0 | 0.4 | 0.982 |
All dietary lipid sources, including menhaden fish oil and the various types of soy-derived lipids with distinct fatty acid profiles, were readily accepted by the juvenile yellowtail and supported normal growth and production performance. This is consistent with the fish oil sparing literature that indicates that nearly any lipid source could be suitable for use in aquafeeds if the essential fatty acid requirements of the targeted species are fulfilled.
In our previous work, we demonstrated the importance of ARA, DHA, and (to a lesser extent) EPA in satisfying the essential fatty acid requirements of California yellowtail, so these were provided directly in the form of lipid supplements to the soy lipid-based feeds. Although the requirements for growth and survival were met, it was expected that changes in dietary lipid source and composition would result in changes in tissue fatty acid profiles. In fact, tissue fatty acid profiles did generally mimic dietary fatty acid composition as reported in the literature.
It is becoming more evident that fatty acid composition of dietary alternative lipid is important. Specifically, the abundance of SFAs vs. unsaturated fatty acids influences the magnitude of tissue fatty acid distortion and it is possible to manipulate tissue fatty acid composition, including LC-PUFA levels, using dietary lipids with certain fatty acid profiles.
Lipids rich in C18 PUFA seems to negatively interfere with LC-PUFA availability and deposition in tissues, whereas those rich in SFAs and/or MUFAs have been described as advantageous in the context of preserving tissue fatty acid composition and enhancing availability of LCPUFA. Although few studies reported no such benefits of SFA- and MUFA-rich lipids, this trend appears to be valid for a number of freshwater and marine taxa.
Additionally, in some circumstances SFA-rich diets appear to substantially reduce dietary requirements for LC-PUFAs and dietary supplementation levels to enhance tissue deposition of these nutrients. This mechanism, in which LC-PUFA availability and deposition are correlated with dietary SFA and MUFA, has been described as the omega-3 or LC-PUFA “sparing effect.”
Fatty acids with shorter chain lengths and lower degrees of unsaturation appear to be preferentially targeted for catabolic shunting. The most abundant fatty acids in SFA- and MUFA-rich lipids used in this study were 18:0 and 18:1n-9, respectively. Presumably a catabolic preference for 18:0 over 18:1n-9 contributed to the underrepresentation of 18:0 in the tissues for (or “resulting in”) at least part of the sparing effect advantage of the SFA-rich feeds over the
MUFA-rich feeds. Regardless of the mechanism(s) responsible, SFAs appear to provide a distinct benefit beyond that associated with MUFAs in terms of conserving tissue fatty acid profiles and LC-PUFA levels.
So far, most studies have evaluated the benefits of dietary SFAs and MUFAs separately. Our study clearly demonstrated the benefits of SFA-rich diets over MUFA-rich diets in the context of preserving tissue fatty acid profile and LC-PUFA deposition. The degree of overall tissue fatty acid distortion was softened by dietary SFA, increasing dietary levels of SFAs increased profile similarity to FISH control diet.
The dietary treatments we tested significantly affected fillet total lipid content. Lower lipid levels were observed in the SFA SOY and LOW PUFA BLEND groups, while the FISH and C18 PUFA SOY groups had the highest lipid levels in fillet. This observation – fish oil-based diets yielding higher total lipid content in fillet compared to alternative and complementary lipid sources – has also been reported in other fish species like Atlantic salmon and white sea bass, but not in others like Florida pompano, European sea bass and cobia.
Seemingly, the effect of dietary fatty acid composition on fillet lipid level might be a species-specific response. The dietary fatty acid profile generally influences tissue lipid class composition, in particular neutral lipids, which seem to mimic the dietary fatty acid composition; whereas, polar lipids are more selective exhibiting a preference for certain fatty acids. Although we did not investigate lipid classes, the lipid class composition of fillet was likely modified due to significant changes in the total lipid content.

Perspectives
Based on results of our study, there is a stronger relationship between dietary levels of PUFAs (negative) and SFAs (positive) and tissue fatty acid profile composition, including LC-PUFA content, than dietary levels of MUFAs.
We also found that SFA-rich lipids maintained tissue profile and produced equivalent or higher fillet levels of LC-PUFAs compared to the LC-PUFA-rich FISH diet, whereas MUFA- and C18 PUFA-rich lipids contributed, to a lesser and greater magnitude, respectively, towards tissue profile distortion at the expense of LC-PUFAs.
Consequently, we propose that dietary SFAs and MUFAs interact antagonistically, that MUFAs are inferior to SFAs in terms of LC-PUFA sparing, and that SFAs are the primary drivers in the LC-PUFA “sparing effect” mechanism.
Authors
-
Artur N. Rombenso, Ph.D.
Corresponding author
Center for Fisheries, Aquaculture and Aquatic Sciences and Department of Zoology
Southern Illinois University
Carbondale, IL 62901 USA;
and Nutrition Laboratory
Institute of Oceanography
Autonomous University of Baja California
Ensenada, Baja California 22860, Mexico
Current affiliation: CSIRO Aquaculture Program
Bribie Island Research Centre
144 North St, Woorim, QLD 4507 AUS[117,97,46,111,114,105,115,99,64,111,115,110,101,98,109,111,114,46,114,117,116,114,97]
-
Jesse T. Trushenski, Ph.D.
Eagle Fish Health Laboratory
Idaho Department of Fish and Game
1800 Trout Road, Eagle, ID 83616 USA
Current affiliation: Riverence,
10414 173rdAve SW
Rochester, WA 98501 USA -
Mark Drawbridge, M.S.
Hubbs-SeaWorld Research Institute
2595 Ingraham Street
San Diego, CA 92109 USA
Tagged With
Related Posts

Aquafeeds
Alternative lipids spare fish oil in rainbow trout feeds
Alternative lipids have achieved varied success in ensuring adequate growth and fatty acid composition in fillets. The authors evaluated rainbow trout raised on diets containing fish oil or a blend of fish and standard or modified lipids varying in fatty acid composition.

Intelligence
Comparing nutritional values of wild and farmed whitefish
A study assessed the nutritional value of farmed vs. wild white-fleshed fishes using hybrid striped bass, largemouth bass and bluegill. Results showed that farmed, white-fleshed fish are as nutritionally valuable – and in some cases, more so – than wild fish.

Aquafeeds
Evaluating dietary fish oil replacement in juvenile Florida pompano
Study evaluated production performance and tissue composition of juvenile Florida pompano fed diets containing fish oil or 25:75 blends of fish oil and various other lipid sources.

Health & Welfare
Long-chain PUFA requirements of juvenile California yellowtail
This study shows that soybean oil can totally replace fish oil in California yellowtail aquafeeds without affecting production performance and reducing the omega-3 fatty acid profile of fish tissues, as long as acceptable levels of ARA and DHA are provided.