Results show cost reduction, optimization potential for economical, sustainable operations
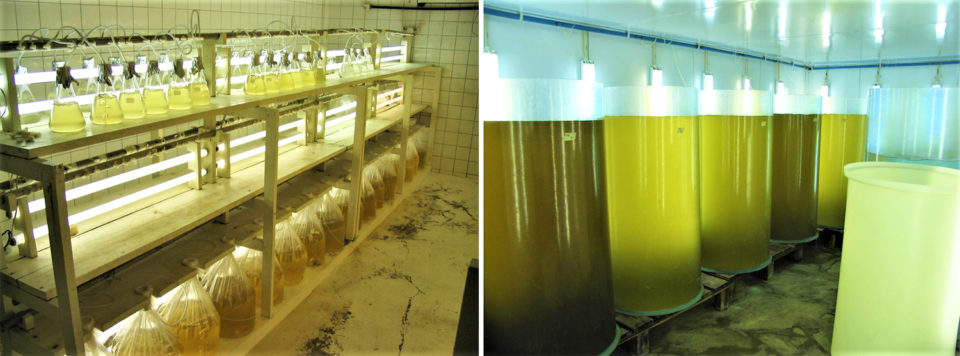
The use of microalgae in aquaculture has increased in the past decade, because of the importance of microalgae as the primary source of nutrition for all stages of filter-feeder bivalves and the larval and juvenile stages of fish and shrimp. The quality of feed is linked to survival and mortality rates of larval stages, development rate, egg viability and other performance parameters of the species cultured.
For a constant supply of fresh microalgae, aquaculture hatcheries generally have an in-house microalgae production facility, generally small in scale and up to 100 square meters in area. In the early 1990s, maintaining a microalgae production facility was estimated by researchers to account for an average of 30 percent, and up to 60 percent of the total budget of aquaculture hatcheries and nurseries, and the cost of microalgae biomass in aquaculture hatcheries was estimated at $50 to $400 (U.S.) per kg dry weight (DW) depending on the applied scale.
Despite the high cost prices, no effort has been described in literature to determine the cost price of microalgae in detail and highlight strategies for cost reduction. Microalgae production systems in aquaculture are typically not selected or optimized for cost efficient production. Most relevant studies available in the literature on microalgae for aquaculture applications focus on the nutritional value of the algae only.
A techno-economic analysis of small-scale algae production facilities – such as the systems used in aquaculture – is not available, as these analyses for microalgae production are typically performed on much larger scales, focusing on the production of bulk chemicals and commodities such as biofuels.
This article – adapted and summarized from the original publication [Oostlander, P.C. et al. 2020. Microalgae production cost in aquaculture hatcheries. Aquaculture, Volume 525, 30 August 2020, 735310] – evaluated the production costs and cost distribution of microalgae production in aquaculture hatcheries, and provides guidelines for cost reduction strategies.
Study setup
We developed a techno-economic analysis for small scale microalgae production facilities (25 to 1500 square meters) to determine the cost-price and cost-distribution of microalgae production facilities in the Dutch aquaculture industry and to identify the most efficient cost reducing strategies. We used data from commercial microalgae facilities in the Netherlands to model reference scenarios describing the cost price of microalgae production with state-of-the-art technology in aquaculture for a biomass production capacity of 125 kg/year.
Commercially available reactors commonly used in aquaculture were compared; tubular photobioreactors (TPBR) and bubble-columns (BC) in two placement possibilities, using artificial light in an indoor facility (AL) and utilizing sunlight in a greenhouse (GH) under Dutch climate conditions.

For detailed information on study design; reactor type and placement; reference scenarios; biomass yield on light; sensitivity analysis; and effect of scale, refer to the original publication.
Results and discussion
The results of cost price and biomass capacity for the reference scenarios in the techno-economic analysis are shown in Fig. 2. The lowest overall production cost for two placement options, greenhouse (GH) and artificial light (AL) is found with the tubular photobioreactor (TPBR) scenarios in both settings.
A cost price of €290/kg ($343.07/kg) is found for the AL-TPBR scenario and €329/kg ($389.20/kg) for the GH-TPBR scenario. These results clearly show a cost price advantage for the TPBR systems over the bubble-column (BC) systems in the reference scenarios. The BC scenarios result in a cost price of €587/kg ($694.40/kg) for the AL-BC and €573/kg ($677.63/kg) for the GH-BC scenarios, respectively. These cost prices are in the order of 100 times higher than numbers described by other researchers but describe algae production on a much smaller scale than number described by these other scientists.
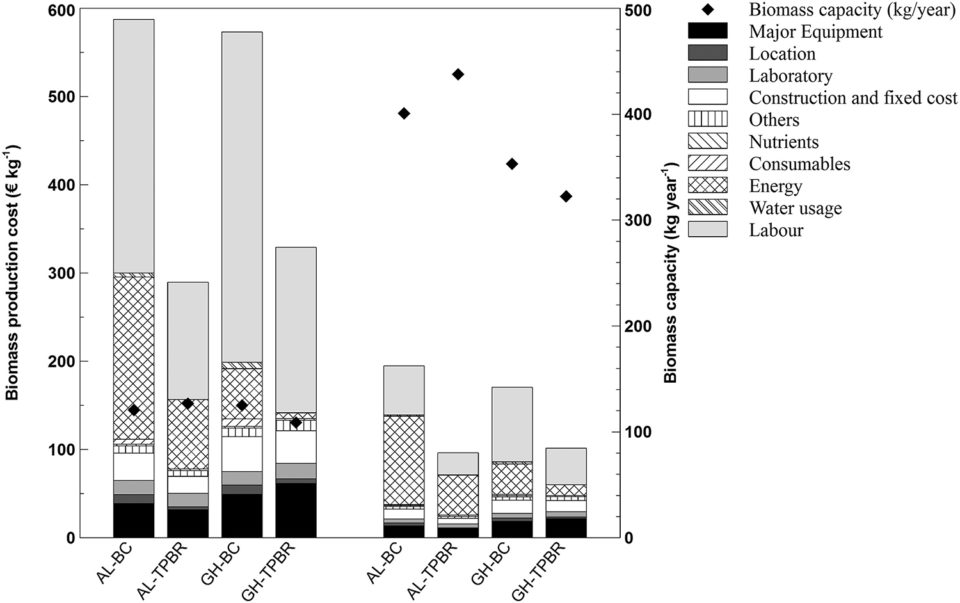
Regarding sensitivity analysis, the results show the effect of each input parameter on the cost price. The results of cost price reduction (in percent from the reference scenarios) are presented in Fig. 3.

The results in Fig. 3 show that additional artificial light has a large impact on the cost price for all systems. A cost price reduction in biomass production is found for all four scenarios with additional artificial light. Doubling the artificial light in AL scenarios doubles the total biomass production per year while adding costs for the lights in both capital expenditure, CAPEX [funds spent by an organization to maintain, buy or improve its fixed assets, such as buildings, equipment, vehicles or land] and operating expenses, OPEX [ongoing costs for operating a business, product or system].
The addition of artificial light can be regarded as an easy to implement and cost-effective method for cost price reduction in existing microalgae production for aquaculture facilities if the reference cost price is higher than the cost of additional light. The further use of highly efficient light sources – such as Light-Emitting Diodes, LEDs [semiconductor light sources that emits light when current flows through it] – will make the use of artificial light even more economically interesting for small scale algae production than is shown in our results.
We only tested the temperature range for the GH scenarios. In the AL scenarios, a constant temperature of the indoor facility is assumed and cooling requirements are based on heat production by the artificial lights. For the GH-BC scenario, reactors are not actively controlled for temperature and a change in the temperature range does therefore not affect the cost price. In the GH-TPBR scenario the temperature of the systems is actively controlled. The larger temperature range results in a reduced cost price, but only by a very small percentage of around 0.36 percent.
The cost price reductions between the different scenarios and the differences observed could be attributed to actual temperature range differences, location (solar input, wind speeds, etc.), electricity cost, reactor geometry and more. The cost reduction in our calculations vs. those of other researchers is smaller due to the much higher cost price for biomass used as reference. Algae growth at temperatures other than the optimal growth temperatures will result in a negative effect on the biomass on light. Therefore, we conclude that to be more cost efficient on these small scales, efforts should target optimizing temperature control to maximize biomass yield on light (Yx,ph), rather than on energy reduction.
The biomass yield on light (Yx,ph) has a large effect on the cost price, as this directly impacts the total amount of biomass produced. The same effect of biomass yield on light is found for all systems: by increasing the biomass yield on light, the total amount of biomass produced is increased without adding costs in our calculations. These results show the importance of operating a microalgae facility using optimized growth parameters and maximizing the biomass yield on light. The value we determined, 0.22 to 0.32 g/mol [a mol is the unit of measurement for amount of substance in the International System of Units, SI] for the monitored systems is much lower than values available in literature
Biomass production cost is a function of the total biomass capacity (kg) and total cost (€, U.S.$) of a production facility. Increasing biomass yield on light should only be the aim if it leads to a larger total biomass production without substantially increasing operational cost. Research focused on understanding the algal species used in aquaculture application and optimizing the applied growth parameters for most cost-efficient production is, therefore, one of the most promising cost reduction methods for small scale microalgae production but requires more effort than other cost reduction strategies.
Labor is the largest cost price component for all reference scenarios, representing between 46 and 65 percent of total cost. More reactor capacity results in a larger labor requirement and therefore a larger effect of labor reduction. In practical applications, reduced labor costs would typically be obtained by the implementation of more automation, for example, for harvesting, for nutrient addition and with artificial light systems, among others, typically linked to higher CAPEX. A breakeven point between additional automation and labor reduction could be determined using the presented model and would be different for each scenario.
Considering seasonal production, the scenarios in greenhouses under the Dutch climate show a very low biomass productivity in the winter months due to low light availability. The scenarios simulated with no production in winter show a reduction of the microalgae production costs of 12.6 percent and 7.9 percent for the GH-BC and GH-TPBR scenarios, compared to production during all days of the year.
Regarding the effects of scale, in an algae production facility it has a large impact on the cost price. The total effect of scale on the cost price for each scenario is compared by comparing scenarios at equal floor area. The results for the effect of scale are summarized in Fig. 4, showing the cost price as a function of total biomass capacity (kg microalgae/year) for each of the scenarios.

The results related to scale are highly dependent on the selected inputs from the starting reference scenario. Therefore, and because it is a parameter with a high impact, the effect of scale was analyzed in more detail for a total of nine scenarios. This analysis shows the potential cost price reduction that could be achieved when producing algae for multiple aquaculture facilities in one centralized algae production facility. Four scenarios show the cost price at different scales for the reference scenarios (solid lines in Fig. 4). The scenarios with combined optimization of the sensitivity analysis (1 through 5) are used for a future outlook on biomass cost price at larger scales (dotted lines in Fig. 4). Additionally, a GH-TPBR scenario using the combined cost reduction methods from the sensitivity analysis is modelled without the addition of artificial light (GH-TPBR-NoAL) in order to indicate the breakeven point for the use of artificial light in a GH-TPBR scenario.
The overall lowest biomass production cost for the scenarios studied is found for the GH-TPBR scenarios not utilizing artificial lights (GH-TPBR-NoAL) on the largest scale (1,500 square meters) producing 6,892 kg microalgae/year at €23.47/kg (US$). The addition of artificial lights on a larger scale under more optimized conditions yields higher biomass cost price while producing much more biomass (11,822 kg/year at €26.35/kg for the GH-TPBR scenario). This shows that when moving to larger scales the addition of artificial light will not always result in a cost price reduction as it does for the small scale. At the 1,500-square-meter scale the cost of artificial light exceeds the relatively low-cost price of biomass, supporting the results as described for the sensitivity analysis on additional artificial light.
Optimizing the scale of a microalgae production facility shows a big potential for cost reduction. The results show that, for the reference scenarios, the cost price could be reduced by 21 to 24 percent for BC scenarios and by 55 to 65 percent for TPBR scenarios by simply increasing the operation from the reference scenarios of 25 to 40 square meters to 250 square meters, and much further when moving up to 1,500 square meters in production area. However, most aquaculture facilities do not require the amount of biomass that is produced at these larger scales. But a specialized algae facility providing biomass for multiple aquaculture applications could produce at a larger scale, reaching much lower production prices than the smaller individual systems.
The scenarios describing the lowest biomass production cost in this comparison, GH-TPBR-NoAL at €23,47/kg ($27.76) and GH-TPBR at €26,35/kg ($31.16), produce 55-100X the biomass capacity as described in the reference scenarios for aquaculture. More realistic near-term prediction can be found in the result for GH-TPBR-Base at 250 square meters. This scenario describes a facility producing 762 kg/year at €123/kg (US$145.46) without further improvements of state-of-the-art technologies other than a scale increase.
Perspectives
Using a techno-economic analysis, we evaluated the cost price of small-scale, industrial microalgae production facilities as presently used in the aquaculture industry. Modelled scenarios based on current commercial standards showed that microalgae biomass cost prices are currently between €587/kg microalgae ($694.66/kg) and €290/kg ($343.20/kg). Two of the most applied commercial systems were compared (bubble-columns vs. tubular reactor systems), showing a significant price advantage for the tubular reactor systems in all tested scenarios
The potential for cost price reduction based on state-of-the-art technology and operation can be significant: our results show that up to 90 percent cost reduction can be achieved from the reference scenarios. From the tested scenarios, the addition of artificial light, increasing biomass yield on light (Yx,ph) and reducing labor requirements showed the largest potential for cost price reduction when not changing the scale of the production facility.
For existing production facilities the easiest strategy for cost price reduction is the addition of artificial light, yielding a cost reduction of 35 to 36 percent for all tested scenarios. Other cost price reducing strategies should focus on higher efficiency of the available production methods, thereby increasing the biomass yield on light. This could be achieved by optimizing growth parameters for the produced strains by optimizing temperature, light, pH, nutrient availability and harvesting strategies in the applied systems. Changes in temperature should focus on improved growth rather than cost reduction for energy consumption.
The size of the facility shows the largest impact on the cost price of microalgae, but a larger scale could be difficult to implement in most existing facilities due to a lack of biomass requirements. A centralized microalgae production facility providing high quality microalgae for multiple aquaculture applications could decrease the cost price by 60 to 80 percent when producing 762 kg/year at €123/kg ($145.56/kg) on a 250-square-meter scale, compared to the 125/kg/year in the reference scenarios. This larger scale is still considered to be a very small production facility but does show a large reduction of overall biomass production cost.
On an even larger scale (1,500 square meters), a cost price of €43/kg ($50.89/kg) is feasible with state-of-the-art technology, and future improvements can reduce this to €26.40/kg ($31.24) on the large 1,500-square-meter scale utilizing TPBR-systems. The large cost reduction that could be achieved in such a facility could have a large impact on aquaculture production processes in the future.
References available in the original publication.
Now that you've finished reading the article ...
… we hope you’ll consider supporting our mission to document the evolution of the global aquaculture industry and share our vast network of contributors’ expansive knowledge every week.
By becoming a Global Seafood Alliance member, you’re ensuring that all of the pre-competitive work we do through member benefits, resources and events can continue. Individual membership costs just $50 a year. GSA individual and corporate members receive complimentary access to a series of GOAL virtual events beginning in April. Join now.
Not a GSA member? Join us.
Authors
-
P.C. Oostlander, Ph.D.
Corresponding author
Bioprocess Engineering, AlgaePARC
Wageningen University
P.O. Box 16, 6700, AA, Wageningen, the Netherlands; and
Research Group Aquaculture
HZ University of Applied Sciences
Edisonweg 4, 4382, NW, Vlissingen, the Netherlands[108,110,46,114,117,119,64,114,101,100,110,97,108,116,115,111,111,46,114,101,116,101,105,80]
-
J. van Houcke, Ph.D.
Research Group Aquaculture
HZ University of Applied Sciences
Edisonweg 4, 4382, NW, Vlissingen, the Netherlands -
R.H. Wijffels, Ph.D.
Bioprocess Engineering, AlgaePARC
Wageningen University
P.O. Box 16, 6700, AA, Wageningen, the Netherlands; and
Faculty of Biosciences and Aquaculture
Nord University
N-8049 Bodø, Norway -
M.J. Barbosa, Ph.D.
Bioprocess Engineering, AlgaePARC
Wageningen University
P.O. Box 16, 6700, AA, Wageningen, the Netherlands
Tagged With
Related Posts

Innovation & Investment
Algae innovators aim to freeze out early-stage shrimp losses
A greenhouse in Belgium believes its innovative shrimp feed product, made from freeze-dried microalgae, packs the necessary nutrients for the crustacean’s most vulnerable life stage: the first three days of its life.

Aquafeeds
Aquaculture Exchange: Giovanni Turchini, Deakin University, part 2
In the second part of our interview with the associate head of research at the School of Life and Environmental Sciences at Deakin University (Australia) he discusses the various alternative sources of omega-3 fatty acids coming on to the market and why research is crucial to advancing aquaculture.

Aquafeeds
Considerations for alternative ingredients in aquafeeds
A key to expanding aquaculture is finding alternative sources of proteins and oils. Supplementing or replacing fish oil in aquaculture feeds with alternative lipid sources – oils seeds, microalgae, insects and others – appears possible as long as essential fatty acid (EFA) requirements are satisfied.

Aquafeeds
Can corn fuel aquaculture’s growth? Veramaris says yes
The benefits of omega-3 fatty acids to human health are well known. Fish need them too. To supplement current supplies from wild-caught fish, one innovative venture is turning to the corn fields of Nebraska – yes, Nebraska – for answers.