Net pens deliver more fish with smaller carbon footprint than tank culture
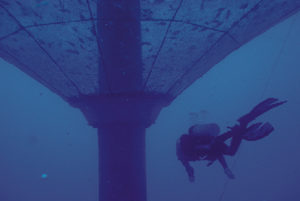
Arguments against net pen fish farms often point to closed containment systems as a solution to environmental impacts on water quality, benthic impacts and wild fish health. But are closed systems actually better in these areas?
Kona Blue has cultured sashimi-grade almaco jack (Seriola rivoliana) in both a land-based pre-commercial production system and an open- ocean fish farm. This presented an opportunity to compare the two grow-out systems in an assessment of several of the salient environmental impacts and other factors, including biological loading and stocking densities, energy use and carbon footprint differential.
During the 2001 to 2004 pilot-scale and test marketing period of Kona Blue’s development, the company operated eight 50-cubic-meter polyethylene-lined steel tanks in a land-based production system at its hatchery/research facility. Offshore operations began in February 2005, and the company now has eight 3,000-cubic-meter submersible net pens at its 36.4-hectare site with more than 30 months of operating experience in the offshore environment.
Biological loading, stocking densities
The production capacities, biological loadings and stocking densities of both land-based and open ocean net pen systems are presented in Table 1. The volume of water and water exchange rates are of course far higher in the offshore system than the land-based system. The mean density of fish in the land-based system was 67 percent higher than offshore, which was needed to defray the higher capital and operating costs for fish on shore. In the offshore net pens, lower densities can be used, as the production capacity is much greater, and operating costs are lower.
Simms, Production capacity and biological loading, Table 1
Land-Based Tanks | Open-Ocean Net Pens |
---|
Land-Based Tanks | Open-Ocean Net Pens | |
---|---|---|
Unit volume (l) | 25,000 * | 3,000,000 |
Total capacity (eight units, l) | 200,000 | 24,000,000 |
Mean density (kg/m3) | 25 | 15 |
Mean standing stock (kg) | 5,000 | 360,000 |
Water exchange (turnovers/hour) | 0.25 | 60 |
Flow rate (l/hour) | 6,250 | 720,000,000 |
Loading (kg/l/hour) | 0.8 | 0.0005 |
Production capacity | 10 mt/year | 720 mt/year |
The health and well-being of the fish in each culture system can only be subjectively assessed. The 1,600 times greater biological loading and greater density in the land-based tanks may have had some less-humane element, but fish in both systems did not display signs of crowding stress. Fish often school densely in net pens, even when there is other available space. The open net pen system, however, is significantly closer to the natural environment and native habitat of the fish.
Offshore, fish experience natural lighting, seasonal changes, tides and currents, and are separated from the offshore environment – and other fish – only by the Kevlar mesh of the net pen. In their 24-meter-diameter pens, suspended in water over 60 meters deep, the fish at the offshore site are more than 30 meters above the substrate.
The land-based tanks, however, were far less natural an environment. The 9-meter-diameter tanks were only 0.9 meters deep, and fish were always close to the tank bottom, with its accumulation of feces and other particulates. The tanks were heavily shaded in 90 percent shade cloth to reduce macroalgal growth. Fish were therefore removed from natural lighting, as well as natural current and tidal movements. The centripetal movement of the water to aid in removal of solids also restricted the ability of the fish to move throughout the tank. Fish often remained generally in one position, oriented into the circular current motion.
Energy use, carbon footprint
The major energy input into the land-based tank culture of the fish was in the pumping of the water. The Natural Energy Laboratory of Hawaii Authority (NELHA), from which Kona Blue leases ocean water and land for its hatchery, pumps water through a common reticulation system that moves around 378 cubic meters per minute. This provides economies of scale similar to a larger commercial farm operation. No additional booster pumps were needed at the Kona Blue site, since the fish in the tanks were raised with unfiltered raw seawater.
The top of the tank water surface was around 5 meters above sea level. In a floating closed-containment system, there would be no head for pumping water to a higher level. However, control of pathogens or parasites required some level of water pretreatment, and additional booster pumps would be needed to move the water through filters to remove the cysts, eggs or other disease vectors. This head pressure would probably be about the same as the Kona Blue tank head height. Pumps at NELHA are all powered by electricity generated by diesel generators.
The major energy input for the offshore net pen system is the boat power required to move feed and farm workers out to the farm site and bring farm workers and harvested product back to shore. The energy inputs and equivalent carbon burden of the offshore and land-based culture systems are presented in Tables 2 and 3.
Sims, Carbon footprint for land-based tank system, Table 2
Land-Based Tanks |
---|
Land-Based Tanks | |
---|---|
Electricity demand (kWh/day) | 64 |
Carbon dioxide produced by electricity generation 0.55 (lbs/kWh) | 0.55 * |
Carbon footprint (mt carbon dioxide/year) | 5.7 |
Production capacity (mt/year) | 10 |
Fish produced/mt carbon dioxide (mt) | 1.74 |
Sims, Carbon footprint for ocean net pens, Table 3
Open-Ocean Net Pens |
---|
Open-Ocean Net Pens | |
---|---|
Diesel consumption (l/day) | 189.27 |
Carbon dioxide produced by diesel engines (lbs/kWh) | 22.40 * |
Carbon footprint (mt carbon dioxide/year) | 200 |
Production capacity (mt/year) | 720 |
Fish produced/mt carbon dioxide (mt) | 3.58 |
This analysis presumed that all other inputs to farm operations – such as shipping feed from the factory, chilling and packing fish, or shipping fish to market – were equal for both land-based and ocean systems. The offshore operation is therefore about twice as efficient per kilogram of product as the land-based operation in terms of energy use and carbon inputs.
In addition, the land-based production system required constant supplementation of oxygen through a dissolved oxygen-monitoring system connected to regulators and a bank of liquid oxygen tanks. The eight tanks at full production consumed, on average, one tank per week, or around 127 cubic meters of oxygen. This equated to 662 liters oxygen per gram of fish produced in the land-based system. In the offshore system, oxygen levels are always close to 100 percent saturation, and no supplemental oxygen is normally provided.
Land or sea?
In this comparison of the two systems, the biological loading and animal density, and therefore the physiological stressors on the fish, were far greater in the land-based tank system than the offshore net pens. Yet the tank system took proportionally more energy and generated a proportionally larger carbon footprint to raise the fish.
In a world that is increasingly energy-hungry, should we be using limited resources to grow fish on land or in closed systems? This is analogous to raising chickens in a submarine: You can do it, but it doesn’t make much sense.
(Editor’s Note: This article was originally published in the March/April 2008 print edition of the Global Aquaculture Advocate.)
Now that you've finished reading the article ...
… we hope you’ll consider supporting our mission to document the evolution of the global aquaculture industry and share our vast network of contributors’ expansive knowledge every week.
By becoming a Global Seafood Alliance member, you’re ensuring that all of the pre-competitive work we do through member benefits, resources and events can continue. Individual membership costs just $50 a year. GSA individual and corporate members receive complimentary access to a series of GOAL virtual events beginning in April. Join now.
Not a GSA member? Join us.
Author
Related Posts

Health & Welfare
10 paths to low productivity and profitability with tilapia in sub-Saharan Africa
Tilapia culture in sub-Saharan Africa suffers from low productivity and profitability. A comprehensive management approach is needed to address the root causes.

Aquafeeds
A look at protease enzymes in crustacean nutrition
Food digestion involves digestive enzymes to break down polymeric macromolecules and facilitate nutrient absorption. Enzyme supplementation in aquafeeds is a major alternative to improve feed quality and nutrient digestibility, gut health, compensate digestive enzymes when needed, and may also improve immune responses.

Health & Welfare
A look at tilapia aquaculture in Ghana
Aquaculture in Ghana has overcome its historic fits and starts and is helping to narrow the gap between domestic seafood production and consumption. Production is based on Nile tilapia.

Intelligence
A motive, and a market, for farmed fish in Mexico
Boasting ample areas for aquaculture and a robust domestic demand for seafood – not to mention its close proximity to the U.S. market – a land of opportunity lies in Mexico. Fish farming is primed to meet its potential south of the border.