Study assesses plankton community in a system growing shrimp and red algae

In intensive shrimp culture systems, the microbial community of bacteria, algae, zooplankton and other microorganisms plays important roles in nutrient cycling, providing nutritional compounds such as fatty acids that are essential to the survival and growth of shrimp.
Traditional systems that integrate the production of shrimp and seaweeds have been proposed to promote the reduction of wastes as well as cyanobacteria and other harmful species due to competition for nutrients during the photosynthetic processes of the seaweed. The authors performed a study at the Sustainable Mariculture Laboratory of the Fisheries and Aquaculture Department of the Rural Federal University at Pernambuco in Recife, Brazil, to assess the plankton community in an integrated biofloc system with Pacific white shrimp, Litopenaeus vannamei, and red algae, Gracilaria birdiae.
Study setup
Once a week over seven weeks, phytoplankton were sampled in three tanks with monoculture of shrimp and nine tanks with integrated biofloc systems containing L. vannamei and Gracilaria algae with wet weights of 2.5, 5.0 and 7.5 kg/m3.
Five days prior to stocking the shrimp and seaweed, water from a matrix tank (total ammonia nitrogen level at 0.2 mg/L, nitrite nitrogen at 0.3 mg/L, nitrate nitrogen at 2.2 mg/L, alkalinity 133.9 mg calcium carbonate/L and total suspended solids at 133.6 mg/L) was mixed and equally distributed to fill 12, 40-L black-plastic tanks to approximately 25 percent of the volume. The remaining 75 percent of the tanks was filled with seawater.
No water exchange was carried out during the experimental period, except for the addition of dechlorinated freshwater to compensate for evaporation losses. The light intensity was kept at about 1,000 lux using a fluorescent lamp with a natural photoperiod. Molasses (40 percent organic carbon) was added once a day as a carbon source to maintain the carbon:nitrogen ratio at 12:1. Hydrated lime was used to maintain alkalinity and pH above 100 mg/L and 7.5, respectively.
Stocking, sampling
The experimental units were stocked with shrimp of 0.34 ± 0.01 g initial weight at a density of 500 shrimp/m3. The shrimp were fed daily at 8 a.m., noon and 4 p.m. with a 40 percent-protein commercial shrimp feed, adjusted in volume daily according to the estimated shrimp consumption, mortality rate and leftover feed.
Samples of G. birdiae biomass were collected at the Pau Amarelo beach in Pernambuco, Brazil, and stored in plastic bags for laboratory analysis. Water was drained from all the samples, and weighed after the material was carefully inspected to eliminate encrusted organisms. Seaweed with reproductive structures, signs of depigmentation and necrosis was discarded.
The seaweed was cultivated in rectangular (20.0 x 6.5 x 2.2 cm) polyvinyl chloride modules placed horizontally in tanks. The rectangular modules also were used in control tanks without seaweed.
Water column respiration and photosynthesis were recorded on weekends during the experiment. Gross and net photosynthesis and water column respiration were measured by the classic dark and light bottle method. Initial and final oxygen concentrations were measured with an oxygen meter.
Once a week, vertical sampling was performed using 600-mL plastic bottles for phytoplankton collection. The water was filtered through a cylindrical-conical net with 15-μ mesh to 15 mL, providing a 40-fold more concentrated sample. The phytoplankton was fixed with formalin, buffered with borax and stored in 10-mL plastic containers.
Results
The shrimp survival rates were all above 89 percent during the 42-day experimental period. The 1.29 average feed-conversion ratio (FCR) and 4.0-g final weight of the shrimp in the integrated biofloc systems were significantly higher (P < 0.05) than the 1.74 FCR and 3.12-g weight in the control group.
The mean gross photosynthesis (0.362-0.437 mg oxygen L/hour), net photosynthesis (-0.223-0.281 mg oxygen L/hour) and respiration (0.416-0.544 mg oxygen L/hour) in monoculture and integrated biofloc system are shown in Figure 1. The gross photosynthesis and respiration positive values and the negative net photosynthesis observed were similar to the results in biofloc systems predominantly associated with heterotrophic microorganisms.
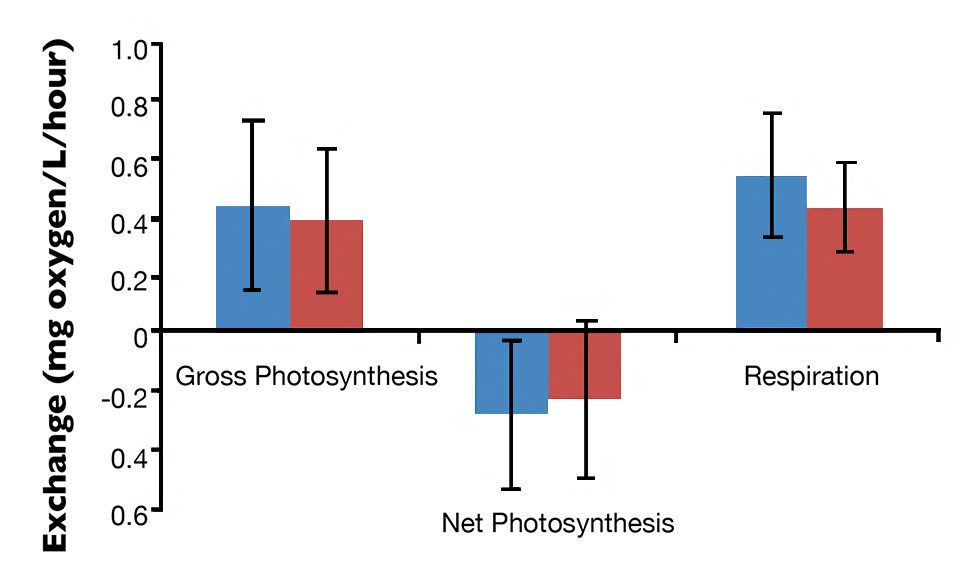
About 61 genera of phytoplankton belonging to the Heterokontophyta phylum were identified. Their densities were about 30,000 cells/mL in both treatments.
Cyanobacteria were the most abundant organisms, followed by Chlorophyta, Heterokontophyta, Euglenophyta and Dinophyta (Figure 2). However, cyanobacteria predominance in integrated biofloc system was less than in the monoculture system. This may be attributed to the increase of organic matter and phosphate in the water, and the competitive advantages of these cyanobacteria over other plankton groups. Therefore, control of the cyanobacteria had been evaluated in a biofloc system with additional diatoms.

About 13 genera of zooplankton belonging to the Rotifera phylum and the Copepoda, Protozoa and Cladocera groups were identified. Their densities were about 1,700/L in both treatments. Fig. 1: Photosynthesis and respiration levels in the water column of monoculture and integrated biofloc systems during a 42-day experiment.
Rotifers were the most abundant zooplankton in the biofloc tanks, followed by copepods, protozoans and cladocerans (Figure 3). The addition of organic carbon in shrimp tanks favored the growth of the zooplankton community, probably due to increased availability of organic matter.

The Rotifera density of 700-790/L, which was higher than in the other zooplankton groups, probably related to the adaptation of these organisms to higher levels of nutrients and solids. Similar results were reported for biofloc or zero-/minimal-water-exchange systems.
Perspectives
In the integrated biofloc system, it seems plankton communities shifted, with decreased amounts of cyanobacteria and increased Heterokontophyta and Chlorophyta. On the other hand, there were fewer Protozoa and increased rotifers and Cladocera. This exchange probably improved the levels of protein and polyunsaturated fatty acids in the biofloc, which contributed to better shrimp growth in the integrated system.
These results suggested additional research should be undertaken to evaluate the biochemical and digestibility attributes of the biofloc.
(Editor’s Note: This article was originally published in the May/June 2015 print edition of the Global Aquaculture Advocate.)
Authors
-
Dr. Alfredo Olivera Gálvez
Departamento de Pesca e Aquicultura
Universidade Federal Rural de Pernambuco
Rua Dom Manoel de Medeiros, s/n Dois Irmãos
CEP: 52171-900
Recife, Pernambuco, Brazil[109,111,99,46,111,111,104,97,121,64,118,105,108,111,95,111,100,101,114,102,108,97]
-
Clarissa Vilela Figueiredo da Silva Campos
Departamento de Pesca e Aquicultura
Universidade Federal Rural de Pernambuco -
Ítala Gabriela Sobral dos Santos
Departamento de Pesca e Aquicultura
Universidade Federal Rural de Pernambuco -
Yllana Ferreira Marinho
Departamento de Pesca e Aquicultura
Universidade Federal Rural de Pernambuco -
Dr. Luis Vinatea
Departamento de Aquicultura
Universidade Federal de Santa Catarina
Florianópolis, Santa Catarina, Brazil -
Luis Otavio Brito
Departamento de Assistência Técnica e Extensão Rural
Instituto Agronômico de Pernambuco
Recife, Pernambuco, Brazil
Tagged With
Related Posts

Responsibility
Giving a lift to tilapia farmers in Myanmar
The U.S. Soybean Export Council’s farm demonstrations, farmer education efforts and formulated feeds are boosting tilapia production in rural areas of Myanmar, where indigenous carp are more commonly raised.

Intelligence
Pangasius aquaculture growing in India
India has strengthened its role as a supplier of seafood to major global markets. Pangasius will continue to be an important contributor to the country’s growing aquaculture production.

Responsibility
Rice-fish integration for high saline, coastal areas of Bangladesh
The Southwestern coastal zone of Bangladesh is agro-based and one of the world’s most populous, poverty-stricken and food-insecure regions. There is an opportunity to diversify and increase productivity by integrating rice with aquaculture during the monsoon season through adoption of water management practices.

Health & Welfare
Acclimating shrimp postlarvae before pond stocking
Shrimp postlarvae acclimation before stocking into the various growout systems (ponds, raceways, tanks) is a critical – and often overlooked, sometimes taken for granted – step in the shrimp culture process. Various water quality parameters should be changed slowly so that the young shrimp have the time to gradually adapt to the new conditions.