Results show algae can be used to manipulate the bacterial community

The stability of a system can be described as the ability to maintain its functions under changing conditions. In the context of recirculating aquaculture systems (RAS), water quality is an important function which relates to stability. Two properties of stability are system resistance (the ability to withstand a disturbance) and resilience (the speed of recovery of a system to its pre-disturbance state). In RAS, disturbances such as pH, oxygen and temperature changes may occur which will consequently affect stability.
Researches have suggested that a stable RAS is linked to its stable bacterial community since bacterial communities plays a central role in maintaining water quality. In addition, it is known that bacteria interact with other microorganisms in the water which may affect the stability of the bacterial community. Therefore, in this study we hypothesized – based on the shared dependency on ammonium by microalgae and nitrifying bacteria – that microalgae could improve the stability of RAS.
Many studies have shown that the association of microalgae with bacteria could lead to a more stable system as is demonstrated by the microalgae-bacterial community in wastewater treatment. And in waste treatment ponds, the existence of the microalgae population is very important for the stability of the symbiotic relationship with aerobic bacteria.
This article – adapted and summarized from the original publication – assessed the role of microalgae on the stability of RAS. We stressed RAS with algae (RAS+A) and without algae (RAS-A) by lowering the water pH from 7 to 4 for three hours. Resistance and resilience of the RAS towards the pH perturbation was calculated by assessing water quality. Additionally, the bacterial communities of RAS+A and RAS-A were compared to determine mechanisms that could explain the RAS stability.
Study setup
The experiment was conducted at the Laboratory of Marine Biotechnology, Institute of Bioscience, Universiti Putra Malaysia. In the experiment, eight recirculating aquaculture systems (RAS) were used. The RAS had been in operation for 10 weeks before this experiment was conducted.
The four RAS with algae (RAS+A) consisted of a fish tank (65 liters), a hydro-cyclone for fecal solids removal, a moving bed reactor with bio-filter media, two tanks units with algae and a sump. The four RAS without algae (RAS-A) had the same configuration as RAS+A except that the tank for algae was filled with water only. Water from the moving bed reactor flowed into two algae tanks, each receiving half of the water flow (3 liters per minute). Water from the algae tanks flowed to the sump from where it was pumped to the fish tank. A periphytic algae (Stigeoclonium nanum) was incorporated in the RAS – it was chosen instead of planktonic algae so that the density of suspended algae in the RAS could be kept sufficiently low to avoid clogging pipes and bio-filters.
The experiment consisted of a period before and after stress. Before the stress (d-1), eight RAS systems were divided over two treatments, RAS with (RAS+A) and RAS without algae (RAS-A). The next day (day 0), two replicates from each treatment were subjected to a stressor (+S) and the other two replicates became the control (no stressor, -S). The stressor that was applied was gradually lowering the pH from 7 to 4 within a period of three to four hours, followed by 3h at pH 4, and thereafter restoring the pH back to 7 within a period of two to three hours. Hence, the whole operation of applying the pH stressor lasted eight to 10 hours in total.
For detailed information on the experimental setup and procedures, microbial analysis (DNA extraction, PCR, and 16 S rRNA metagenomic), data processing and statistical analysis, refer to the original publication.
Results and discussion
There were no significant differences of total ammonia nitrogen (TAN), nitrite-nitrogen (NO2-N) and nitrate-nitrogen (NO3-N) between the sampling locations. TAN concentrations increased in all systems immediately after the stressor was applied (Fig. 1a). Water discharge was performed to control the level of TAN in stressed systems. However, the same water discharge procedure must also be done to control treatment (non-stressed RAS). Water discharge might cause bacterial wash-out and affected nitrifying bacteria. This might be the reason of TAN increased in control treatment after day 7.
Significant differences of TAN concentrations were explained by the factors algae, stressor and day, but not by sample location. In RAS, ammonium may be removed via three processes; conversion to nitrite and subsequently to nitrate through nitrification, immobilization in bacterial and archaeal biomass, and uptake by algae. Since the experiment did not distinguish which process had caused the reduction of TAN in the RAS, apparent TAN conversion is the term used to describe the process. Apparent TAN conversion rate (Fig. 1b) was significantly affected by the factor stressor. Meanwhile, significant differences of nitrite concentrations were explained by the factors algae, stressor and day, but not by sample location.

Results showed that lowering the pH in RAS from pH 7 to pH 4 and maintaining it for three hours disrupted the function of the bacterial communities in the RAS+A and RAS-A as indicated by the deteriorated water quality following the stress application.
Resistance towards the acute pH drop for RAS+A and RAS-A was not significantly different neither for apparent TAN nor for nitrite conversion. The same result was found for the resilience for TAN conversion. However, the resilience for nitrite conversion was significantly higher for the RAS+A than for RAS-A. Therefore, we concluded that the efficiency of ammonium conversion was not different in both treatments.
However, since the nitrite and nitrate concentrations were significantly lower in RAS+A than in RAS-A this might indicate that algae could have absorbed some ammonium, thus less ammonium was available for nitrification, subsequently less ammonium was converted to nitrite and nitrate. Additionally the resilience for nitrite conversion was significantly higher in the RAS+A than RAS-A, indicating that algae had a positive effect on RAS water quality.
Regarding the assimilation rate of ammonium by algae, our results showed that 580 mg algal biomass in the experiment might have assimilated 37 mg ammonium, which was approximately 2.5 percent from the TAN produced by the RAS. When the microbial community was stressed, the uptake of ammonium by algae might stabilize the system and contribute to lower nitrite in RAS+A than RAS-A.
The results from this study strongly suggested that algae influenced the bacterial composition and functions in the RAS as the effect of algae was also observed on day 20 after stress. The ordination of the bacterial communities showed that bacterial communities from RAS+A were separated from the bacterial communities of RAS-A. When bacterial communities were compared between factors algae, location and stressor, the results showed that there were significant differences of bacterial communities for all factors.
For d-1, the dissimilarity between RAS+A and RAS-A was 34 percent, and on d20 it was 44 percent. Fifteen operational taxonomy units (OTUs; an operational definition used to classify groups of closely related individuals) contributed to the top 10 percent from the total dissimilarity between the treatments (Fig 2). Mycobacterium sp. and Novosphingobium sp. were the two groups that were consistently higher in RAS-A than RAS+A on d-1 and d20 after stress. Meanwhile, Microbacteriaceae, Xanthomonadaceae and Verrucomicrobiaceae were found consistently higher in RAS+A than RAS-A on d-1 and d20 after stress.
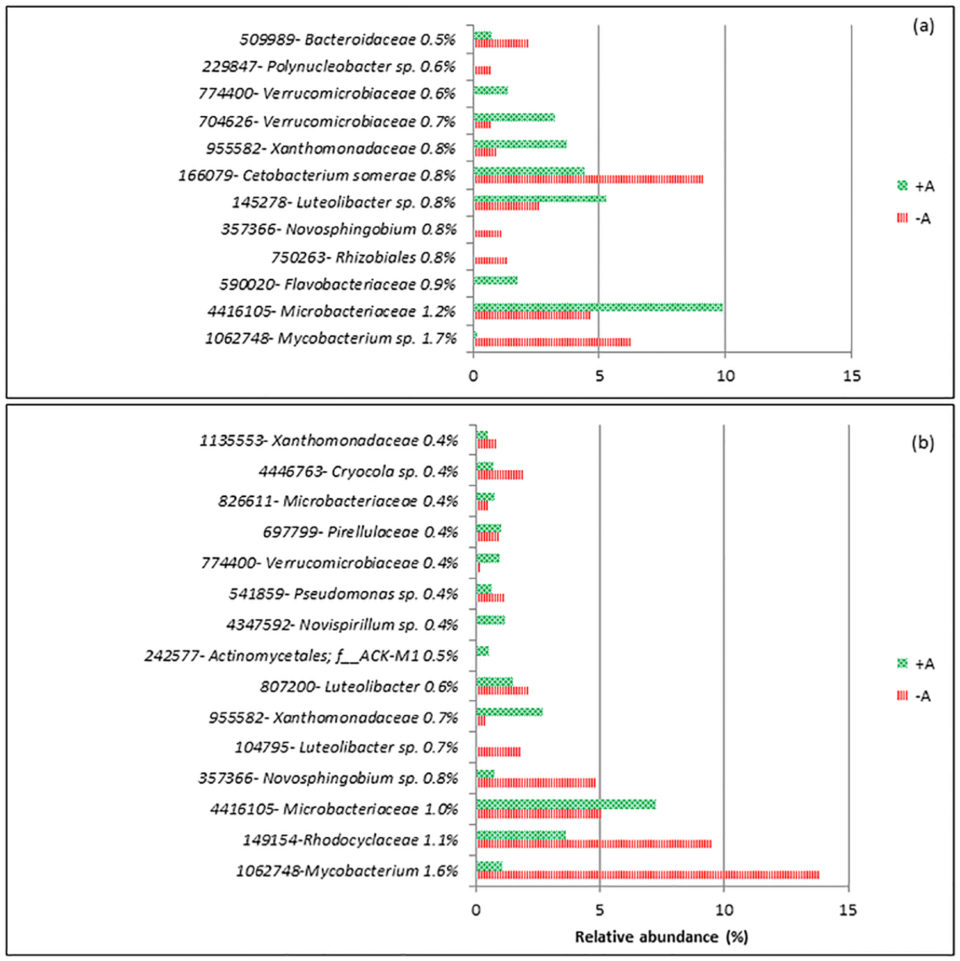
From the results, most of the discriminant bacteria that contributed to the differences between RAS+A and RAS–A were heterotrophic bacteria. This could mean that the different bacterial composition might be caused by the dynamics of organic nutrients in the system. This is very plausible since xenobiotic (any foreign substances or exogenous chemicals) degradation and metabolism was the highest discriminant function between RAS+A and RAS-A. Xenobiotic compounds are generally known as chemicals that are not natural to the environment and are regarded as environmental pollutants.
Bacteria that were more dominant in the RAS+A had the ability to degrade organic nutrients originated by microalgae, and our data showed that the presence of algae stimulates bacterial species which metabolize organic compound released by the algae.
We determined Nitrosomonadaceae and Nitrospira as bacteria involved in autotrophic nitrification; and for heterotrophic nitrification and denitrification, Rhodococcus, Chryseobacterium, Bacillus, Acinetobacter and Pseudomonas were the groups of bacteria involved (S6 Fig). The presence of Nitrosomonadaceae was almost negligible in all RAS. More changes of these bacteria occurred in RAS-A than RAS+A. However, the relative abundance of these bacteria was not significantly different between RAS-A and RAS+A. These bacteria count about 3.5 to 10 percent from the total bacterial abundance and their presence was not affected by algae.
Bacteria which were affected by the algae were mostly from the heterotrophic group. It was expected that the algae concentration was too low to reduce TAN availability for nitrification to measure effects. Therefore, in the future an experiment which will allow a higher immobilization of ammonium by algal biomass should be conducted to be able to measure algae effect on nitrifiers.
Perspectives
Results of this study showed that RAS with and without algae had the same resistance and resilience in restoring to pre-stressor maintenance of low ammonium levels after an acute pH perturbation. Algae supported RAS in keeping the nitrite concentration low before and after the perturbation.
In this regard, we conclude that RAS+A had a better stability than RAS-A. Algae influenced the bacterial community composition in the RAS causing more algal-associated bacteria species to be found in the RAS+A. This suggests strongly that algae can be used to manipulate the bacterial community in RAS.
Now that you've finished reading the article ...
… we hope you’ll consider supporting our mission to document the evolution of the global aquaculture industry and share our vast network of contributors’ expansive knowledge every week.
By becoming a Global Seafood Alliance member, you’re ensuring that all of the pre-competitive work we do through member benefits, resources and events can continue. Individual membership costs just $50 a year. GSA individual and corporate members receive complimentary access to a series of GOAL virtual events beginning in April. Join now.
Not a GSA member? Join us.
Authors
-
Norulhuda Mohamed Ramli, Ph.D.
Aquaculture and Fisheries Group
Wageningen University
Wageningen, The Netherlands; and
Department of Biological and Agricultural Engineering, Faculty of Engineering
Universiti Putra Malaysia
Serdang, Selangor, Malaysia -
Christos Giatsis, Ph.D.
Aquaculture and Fisheries Group
Wageningen University
Wageningen, The Netherlands -
Fatimah Md Yusoff, Ph.D.
Laboratory of Marine Biotechnology, Institute of Bioscience
Universiti Putra Malaysia
Serdang, Selangor, Malaysia; and
Department of Aquaculture, Faculty of Agriculture
Universiti Putra Malaysia, Serdang, Selangor, Malaysia -
Johan Verreth, Ph.D.
Aquaculture and Fisheries Group
Wageningen University,
Wageningen, The Netherlands -
Marc Verdegem, Ph.D.
Aquaculture and Fisheries Group
Wageningen University
Wageningen, The Netherlands
Tagged With
Related Posts

Innovation & Investment
A review of unit processes in RAS systems
Since un-ionized ammonia-nitrogen and nitrite-nitrogen are toxic to most finfish, controlling their concentrations in culture tanks is a primary objective in the design of recirculating aquaculture systems.

Intelligence
RAS in the USA: Fad or future?
A rash of large-scale, land-based recirculating aquaculture systems (RAS) are planting their flags on U.S. soil, even though it will take several years and hundreds of millions of dollars of investment before they produce their first sellable fish.

Innovation & Investment
Is RAS the game-changer that Europe’s aquaculture sector needs?
The impact that recirculating aquaculture systems, or RAS, will have on European production remains to be seen, but the general vibe is positive.

Aquafeeds
Biofloc and clear-water RAS systems: a comparison
This study compared two types of indoor, shrimp culture systems: clear-water RAS and biofloc systems. Clearwater RAS had the edge in water quality, but shrimp in the biofloc treatment had a higher feed conversion ratio.