Ammonia may be used by heterotrophic bacteria
Ruminant nutritionists focus on nurturing healthy microflora populations within the first stomach or rumen of cud-chewing mammals like cattle, sheep, and goats. By managing the rumen, nutritionists are maximizing feed utilization by the animals. Likewise, healthy microflora populations within aquaculture systems serve as a type of natural rumen or bioreactor, which increases utilization of feed nutrients by cultured animals. Bacteria play an important role in the dynamics of nutrients in aquaculture production systems. Because of the importance of nitrogen as a nutrient and metabolite, we will discuss the role of bacteria on nitrogen dynamics.
Nitrogen dynamics
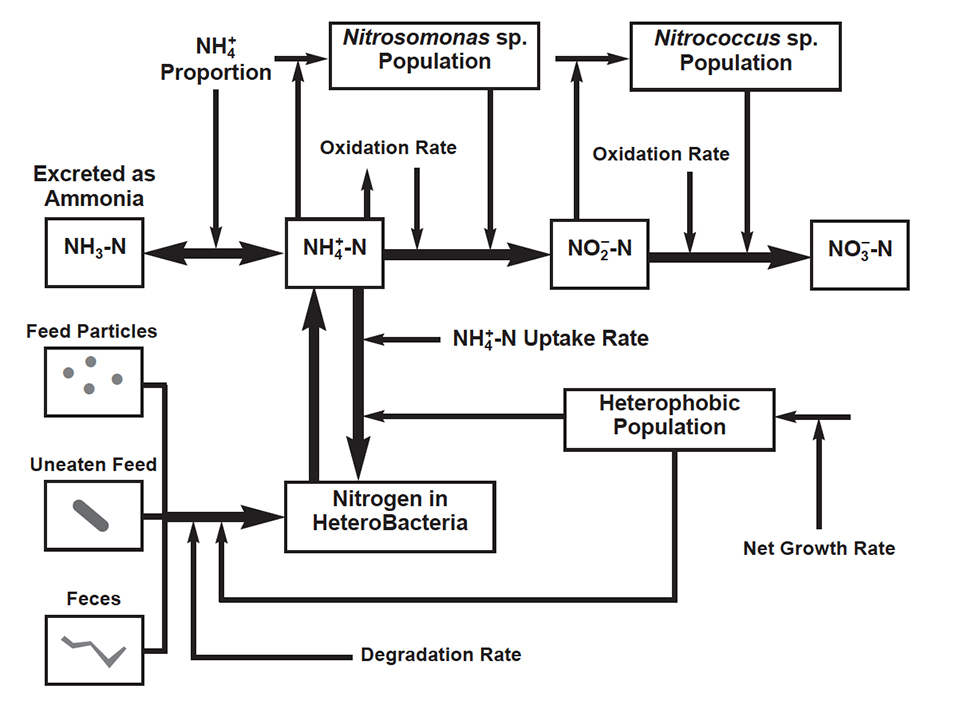
Nitrogen is the main component of proteins, short-chain peptides and amino acids, which in turn are principal components of most artificial and natural feeds used in aquaculture. Following protein consumption and degradation, cultured aquatic species excrete ammonia (NH3), which at high levels is toxic to aquaculture species. In water, ammonia establishes an equilibrium between its toxic form (NH3) and the non-toxic ammonium ion (NH4 +). This equilibrium is mainly dependent on water temperature and pH. Ammonium may be used as a nitrogen source by phytoplankton, algae, plants, and by a group of bacteria called heterotrophs. It may also be used as a source of energy by certain chemoautotrophic bacteria, called nitrifiers.
Until recently, phytoplankton was assumed to be responsible for most of the ammonium uptake, but recent evidence suggests that heterotrophic bacteria are significant users of ammonium in aquaculture systems. Bacteria are efficient scavengers of nutrients and may successfully compete with phytoplankton for uptake of ammonium ions. Some studies indicate that heterotrophs may use up to 50 percent of the total ammonium dissolved in water. However, heterotrophic bacterial growth and demand for nitrogen appear to be higher than maximum ammonium uptake rates, indicating that they also use other sources of nitrogen, such as organic compounds. These could include uneaten feed pellets, disintegrated feed particles, and animal excreta. Most of this organic matter is decomposed aerobically, that is by heterotrophs that consume oxygen. Aerobic heterotrophic bacteria are distributed throughout the water column and sediment surface. These bacteria decompose organic substrates for growth and energy and they utilize ammonium as a building block for protein synthesis.
Decomposition rates depend mainly on various environmental factors and organic substrate quality. Temperature and oxygen are the main environmental determinants of decomposition rate. Organic substrate quality depends upon the type of carbon and energy sources and the nutrients. Carbon sources differ widely, from easily degradable (e.g., sugars) to more resistant compounds (e.g., lignin). Deficiencies in any nutrient required for heterotrophic bacteria could limit decomposition, but in natural ecosystems nitrogen is generally the limiting factor. This situation is reversed in some aquaculture systems, where use of high protein diets actually results in an excess of nitrogen. Considering the key role of nitrogen, the relation between the amount of carbon and the amount of nitrogen, known as the carbon:nitrogen (C:N) ratio, has been used frequently to indicate the quality of organic substrates. For example, two feeds with the same amount of carbon but different amounts of protein, and consequently different levels of nitrogen, will result in different C:N ratios. The feed with more protein will have the smaller C:N ratio.
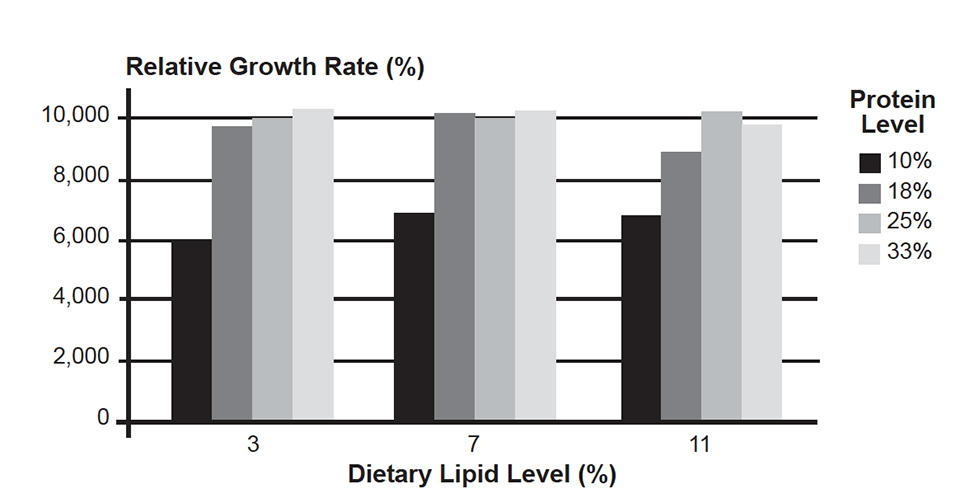
Ammonium regeneration
Part of the nitrogen utilized by heterotrophic bacteria may be regenerated or remineralized back to the water in its inorganic form of ammonium, but this depends upon two conditions. First, the nitrogen source must come from an organic substrate. Second, the C:N ratio of the organic substrate must be less than that of the bacterial biomass. The regeneration of ammonium also varies depending on whether the bacterial population is growing or stationary. When the bacterial population is growing (reproduction rate is higher than death rate), regeneration of ammonium increases with decreasing substrate C:N ratios. When the bacterial population is stationary, regeneration of ammonium increases with increasing substrate C:N ratios, due to concomitant losses in the population itself. No net regeneration occurs when the C:N ratio is greater than 10.
Nitrogen cycling
Ammonia produced by the cultivated species and by heterotrophs may be used by phytoplankton and by a special group of bacteria, the nitrifying bacteria. These bacteria are the only ones that can obtain energy for growth by oxidizing ammonia to nitrate. However, nitrifying bacteria are inefficient – when compared to heterotrophs – in converting available energy to cellular material. For example, Nitrosomonas generates only 1 to 4 percent and Nitrobacter 3 to 10 percent of the cell material that might be expected from a heterotroph with the same amount of available energy.
Bacterial nitrification of ammonia to nitrate is beneficial because nitrate is not toxic for cultivated species. This process occurs in two consecutive steps, each one involving two different groups of nitrifying bacteria. In the first step, aerobic nitrifying bacteria such as Nitrosomonas and Nitrosococcus oxidize ammonia to nitrite. These bacteria are entirely dependent on the oxidation of ammonia for the generation of energy for growth, and depend on adequate oxygen levels. Nitrite may also be toxic to cultivated species.
The second step is the oxidation of nitrite to nitrate, performed by facultative bacteria such as Nitrococcus and Nitrospira. These bacteria are different because they can also use organic compounds for the generation of energy for growth. In contrast to ammonia oxidizers, some nitrite-oxidizing bacteria can grow under low oxygen conditions. Nitrification is affected by many factors in aquatic systems, including temperature, pH, alkalinity, microbial population, and ammonium concentration. The final product of nitrification is nitrate, which may be used by hetetrophic bacteria as a nitrogen source.
Ammonia oxidation to nitrate increases with increasing ammonia concentration until a maximum is reached, which can be mathematically predicted. The rate of nitrification is directly proportional to the growth of nitrifying bacteria populations, which also may be predicted mathematically.
Conclusion
In summary, cultured species excrete ammonia, which may become toxic to them at high levels. However, ammonia may be used by heterotrophic bacteria, which may also use the nitrogen in organic compounds, such as uneaten feed and feces, leading to regeneration of ammonium. Ammonia excreted by cultivated species and regenerated by heterotrophic bacteria may also be used by nitrifying bacteria. These bacteria oxidize ammonia to nitrite, which can become toxic at high levels, and finally to nitrate, which in turn may be used by heterotrophs.
These dynamic interactions are important when considering bacterial management strategies in aquaculture systems. For example, increasing protein in feeds could lead to better growth of the cultured species, but also to a higher regeneration of ammonium by heterotrophic bacteria if C:N ratios are not considered. This becomes more important in recirculating and in low or zero water exchange aquaculture systems stocked at high densities.
We are currently developing a computer simulation model that integrates relationships described here with nutritional and physical characteristics of feeds and feeding strategies. The objective is to better understand the role of bacteria in the nitrogen dynamics of shrimp aquaculture systems. This model will provide further insights on development of eco-efficient aquaculture systems, which are characterized by: maximum nutrient harvestability, maximum growth rate of culture organisms, and minimum land, water, and energy usage per unit of harvestable biomass. Just as ruminant nutritionists manage the microflora of the rumen, aquaculturists may benefit from managing microflora within ponds to reduce nutrient levels in feeds, reduce water requirements, and improve the environment of the cultured species.
(Editor’s Note: This article was originally published in the April 2000 print edition of the Global Aquaculture Advocate.)
Now that you've finished reading the article ...
… we hope you’ll consider supporting our mission to document the evolution of the global aquaculture industry and share our vast network of contributors’ expansive knowledge every week.
By becoming a Global Seafood Alliance member, you’re ensuring that all of the pre-competitive work we do through member benefits, resources and events can continue. Individual membership costs just $50 a year. GSA individual and corporate members receive complimentary access to a series of GOAL virtual events beginning in April. Join now.
Not a GSA member? Join us.
Authors
-
Ruby Montoya, Ph.D.
System Modeling Specialist and Consultant
Ciudadela Los Ceibos
Av. Segunda #321 y Calle Décima
Guayaquil, Ecuador -
Mario Velasco, Ph.D.
Director of Nutrition and Research
ENACA
P.O. Box 09-04-4344
Guayaquil, Ecuador[99,101,46,116,101,110,46,97,117,99,101,64,111,99,115,97,108,101,118,109]
Tagged With
Related Posts

Health & Welfare
Antibiotic-resistant bacteria, part 2
The development of antimicrobial resistance genes in human pathogens as a consequence of exposure to antibiotics in aquaculture is widely documented. Reports implicate foodborne antibacterial-resistant pathogenic bacteria in human disease.

Health & Welfare
Dietary strategies for disease management in the post-antibiotic era
Policies to limit antibiotic use spurred interest in alternative strategies, such as dietary supplementation with immunostimulants and other compounds.

Health & Welfare
Pathogenic Vibrios in shellfish
It is important to understand the distribution of pathogenic bacteria in the marine environment to predict potential health concerns transmitted by seafood.

Aquafeeds
Varied feed additives improve gut, animal health
There is increasing evidence that natural feed additives can have beneficial effects on aquaculture animals by supporting well-balanced gut microflora and improving gut health.