Maintaining good sediment quality is important in pond aquaculture

The bottoms of new ponds usually are low in organic matter concentration because the native top soil is removed during construction. During aquaculture, sedimentation increases the organic matter concentration in bottom soil. Particles originating from mineral soil and organic matter – especially uneaten feed, feces, and the remains of organisms – that do not float or dissolve in pond water settle to the bottom.
Most fish and shrimp farmers realize that maintaining good sediment quality is important in pond aquaculture, but seldom understand the biogeochemical processes that occur in sediment.
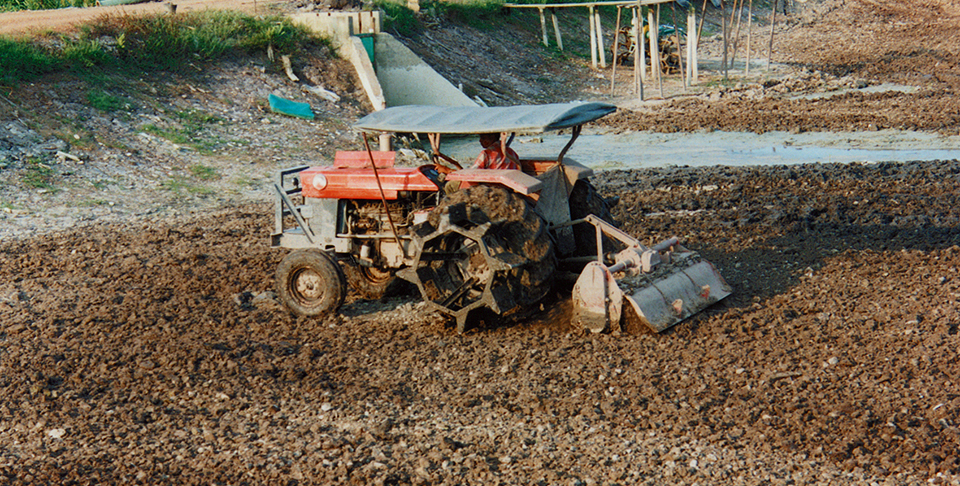
Organic matter composition
Bacteria and other organisms in sediment decompose organic matter, but initially, the rate of input exceeds the rate of decay. In pond soil that receives a fairly constant input of organic matter, organic matter concentration increases for a few years until the rates of organic input and decay reach an equilibrium. At equilibrium, the sediment seldom contains more than 2 to 3 percent organic carbon or 4 to 6 percent organic matter.
Organic matter consists of readily decomposable (labile) and slowly decomposable (refractory) compounds. The labile compounds decompose within days or weeks, while refractory compounds require many months or years to decompose. In aquaculture ponds, the freshly settled organic matter from uneaten feed, feces, and dead plankton is largely labile, but also contains a refractory component.
The oxygen demand of sediment results mainly from the labile fraction of freshly deposited organic matter. It is important to recognize that a comparatively small input of labile organic matter to bottom soil of low organic matter concentration can cause anaerobic conditions at the sediment surface. For example, the accumulation of uneaten feed can cause localized problems with sediment quality in a new pond never used for aquaculture.
Oxygen consumption
To understand what happens when organic matter decomposes in pond bottoms, consider a small, sealed container filled with fresh, aerobic sediment. The water in the pores of the sediment initially contains dissolved oxygen, and aerobic microorganisms use molecular oxygen to transform organic matter to carbon dioxide and water. After a while, the microorganisms deplete the dissolved oxygen.
In the absence of molecular oxygen, fermentation begins. Microorganisms capable of fermentation degrade complex organic matter to carbon dioxide and simple carbon compounds such as alcohols, aldehydes, and ketones. Because fermentation does not mineralize organic matter completely to carbon dioxide, it is not as effective as aerobic respiration in decomposing organic matter.
Instead of molecular oxygen, anaerobic chemotrophic bacteria remove oxygen from inorganic compounds to decompose organic matter to carbon dioxide and water. After oxygen is depleted in sediment pore water, denitrifying bacteria remove oxygen from nitrate and nitrite and use it in respiration. Nitrogen gas is released as a metabolite.
Once nitrate and nitrite are depleted by denitrification, other bacteria remove oxygen from iron and manganese oxides for respiration and produce ferrous and manganous compounds as metabolic byproducts. After these compounds are depleted, some bacteria can remove oxygen from sulfate for use in respiration. Sulfate is transformed to sulfide in the process.
Chemotrophic bacteria often use simple carbon compounds produced in fermentation as carbon sources, rather than more complex organic matter. Finally, carbon dioxide that accumulates in sediment as a result of organic matter decomposition can be a source of oxygen for methane-producing bacteria.
Sequential microbial reactions
In our example container, there was a sequential series of microbial reactions because the sediment sample was confined without a source of molecular oxygen. In ponds the same processes occur, but molecular oxygen that continually diffuses into sediment is used by aerobic microorganisms. Usually, there is no dissolved oxygen in the pore water below a depth of a few millimeters.
Instead of being sequential in time, the reactions stratify within the sediment. Denitrification occurs in the top of the anaerobic layer. Below this, layers contain ferric and manganic compounds, sulfate, and finally carbon dioxide as oxygen sources for microbial decomposition. Fermentation can occur anywhere within the anaerobic zone.
Redox potential
Oxidation-reduction (redox) potential is a measure of the relative degree of oxidation or reduction of substances. Water saturated with dissolved oxygen has a redox potential around 560 mv, while water with only 1 milligrams per liter dissolved oxygen has a redox value above 500 mv. As oxygen is used up in decomposition, redox potential declines. In anaerobic pond sediment, the redox potential declines drastically with increasing depth. The redox potential can be 0 mv or even negative in highly reduced sediment.
The different microbial processes by which organic matter is degraded occur at specific ranges in redox potential (Fig. 1). As long as dissolved oxygen is 1 milligrams per liter or more, the redox potential is high and organic matter degrades aerobically. However, when the oxygen is used up, the redox potential declines and aerobic bacteria no longer decompose organic matter.
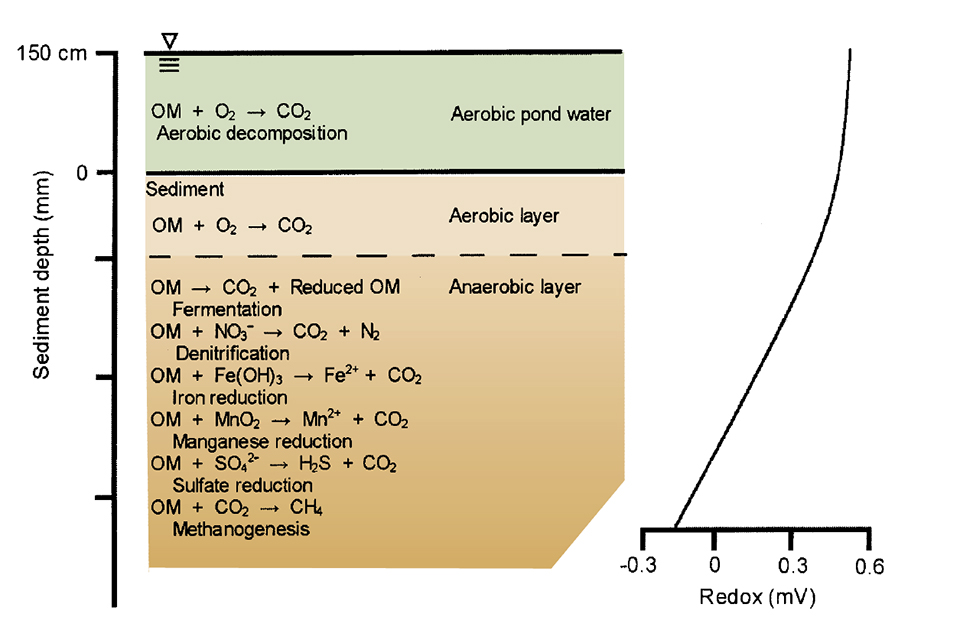
Denitrification begins at a redox of about 300 mv. At around 200 mv, nitrate is depleted, and iron and manganese compounds can be used as oxygen sources. The redox potential will be about 0 mv when sulfate reduction and methanogenesis begin.
Visual difference
Because redox potential changes rapidly over minute vertical distances in sediment, it is difficult to measure and impractical for use in pond management decisions. Nevertheless, one does not need to measure redox potential to determine if sediment is aerobic or anaerobic.
Aerobic and anaerobic sediment differ in appearance. Aerobic sediment is light brown, yellow, gray, or some other natural soil color. Sediment becomes darker when oxygen is depleted, and anaerobic sediment is often gray or black as the result of ferrous iron.
Pond sediment management
Many metabolic wastes in anaerobic sediment, and especially hydrogen sulfide, are toxic to aquaculture species. Thus, it is desirable to maintain an oxidized layer at the sediment-water interface to prevent the compounds from entering the water.
The best way to assure that the sediment surface remains aerobic is to manage ponds to prevent dissolved oxygen concentrations from falling below 3 milligrams per liter and assure good water circulation over pond bottoms. The application of nitrate to pond water also can help prevent low redox potential at the sediment-water interface by supporting denitrification.
When ponds are drained for harvest, bottom soils should be allowed to dry, crack and naturally aerate. This process accelerates the decomposition of the labile organic matter remaining from the previous crop. Also, reduced compounds in the soil are oxidized. Ferrous iron oxidized to ferric iron and sulfide converted to sulfate serve as oxygen sources for microorganisms during the next crop.
Microorganisms decompose organic matter most effectively at pH 7 to 8, so acidic soils should be limed. Also, tilling of pond bottoms enhances aeration. Where soils cannot be dried completely, nitrate fertilizer can be applied as a soil oxidant.
(Editor’s Note: This article was originally published in the October 2004 print edition of the Global Aquaculture Advocate.)
Now that you've finished reading the article ...
… we hope you’ll consider supporting our mission to document the evolution of the global aquaculture industry and share our vast network of contributors’ expansive knowledge every week.
By becoming a Global Seafood Alliance member, you’re ensuring that all of the pre-competitive work we do through member benefits, resources and events can continue. Individual membership costs just $50 a year. GSA individual and corporate members receive complimentary access to a series of GOAL virtual events beginning in April. Join now.
Not a GSA member? Join us.
Author
-
Claude E. Boyd, Ph.D.
Department of Fisheries and Allied Aquacultures
Auburn University
Alabama 36849 USA[117,100,101,46,110,114,117,98,117,97,64,49,101,99,100,121,111,98]
Tagged With
Related Posts

Responsibility
A look at various intensive shrimp farming systems in Asia
The impact of diseases led some Asian shrimp farming countries to develop biofloc and recirculation aquaculture system (RAS) production technologies. Treating incoming water for culture operations and wastewater treatment are biosecurity measures for disease prevention and control.

Health & Welfare
A study of Zoea-2 Syndrome in hatcheries in India, part 1
Indian shrimp hatcheries have experienced larval mortality in the zoea-2 stage, with molt deterioration and resulting in heavy mortality. Authors investigated the problem holistically.

Health & Welfare
A study of Zoea-2 Syndrome in hatcheries in India, part 2
Indian shrimp hatcheries have experienced larval mortality in the zoea-2 stage, with molt deterioration and resulting in heavy mortality. Authors considered biotic and abiotic factors. Part 2 describes results of their study.

Responsibility
Advancing shrimp farm technologies support greater efficiencies, sustainability
Todays' shrimp farm technologies include biosecurity protocols, stocking densities according to pond design and aeration levels, and best practices for pond preparation and feed and water management.