Vaccine development, field experiences in Asia
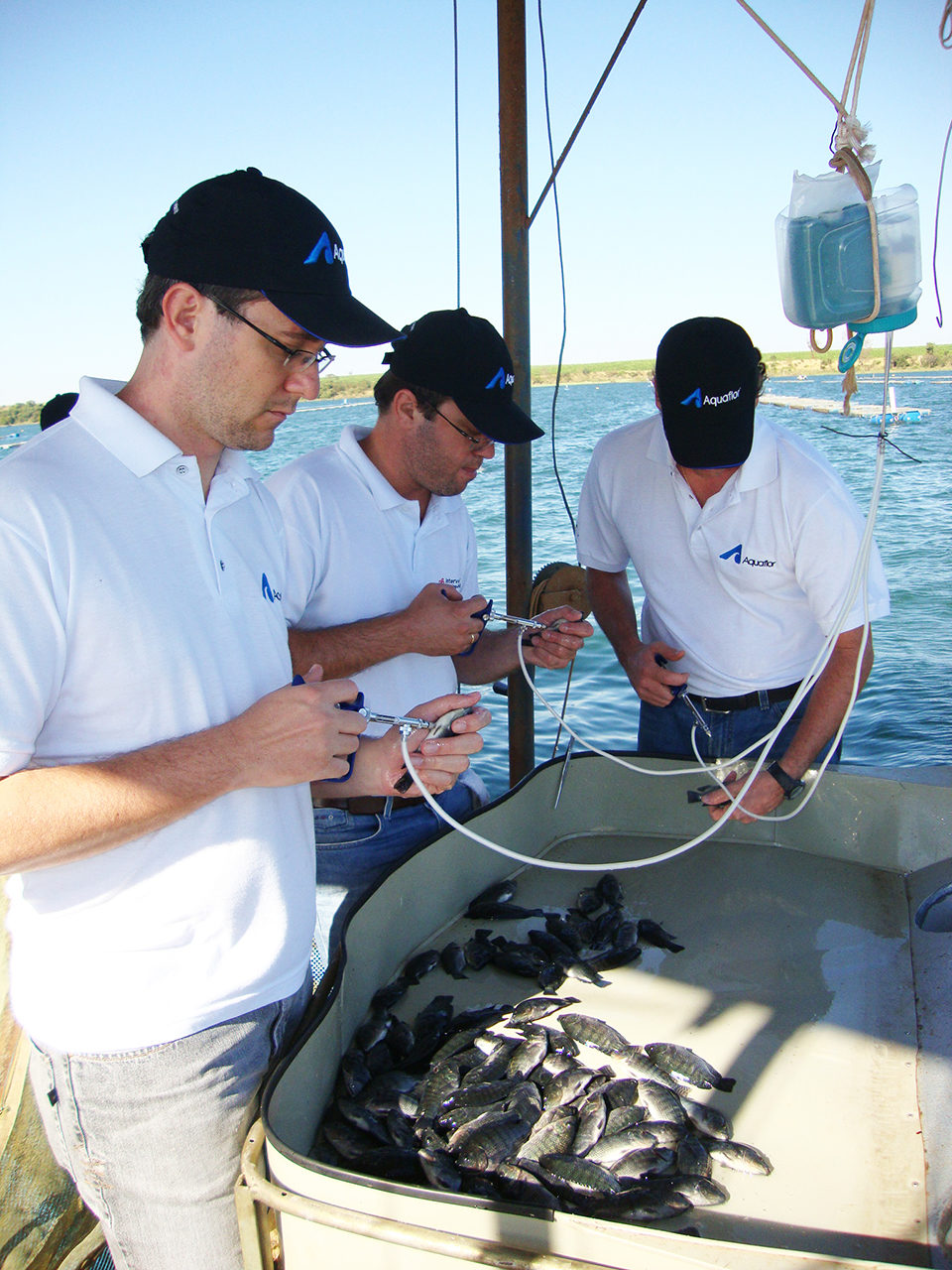
Global production of tilapia has nearly tripled since the beginning of the decade. No other fish species has displayed such aggressive and sustainable growth, year after year.
However, the cost of raw materials is increasing, which is increasing the cost of production and reducing profit margins. Further advancements in production efficiency are required to improve profitability. This trend is driving the aquaculture industry toward consolidation and intensification – a situation that fosters the emergence of production diseases, including several that affect tilapia.
Tilapia diseases
Experience has shown that intensive fish-farming operations can suffer from six to eight major production diseases that must be prevented or controlled. Four major bacterial disease pathogens have been identified in tilapia: Streptococcus agalactiae, S. iniae, Flavobacterium columnare and Francisella species. One viral disease, iridovirus; and two major groups of parasites, Monogenean species such as Gyrodactylus and Protozoan species such as Trichodina, have also been found.
Their prevalence and severity depend on many environmental factors, such as geographical location, culture system, farming intensity, water salinity and temperature. Biological factors such as age, genetics, nutrition and stress also play roles in diseases.
Streptococcus: established pathogen
By far the most important diseases economically are those due to Streptococcus species. In many instances, streptococcosis does not contribute the highest mortality, but it kills large fish and, as a consequence, heavily affects feed-conversion ratios, reduces marketable product and decreases production and processing efficiency.
In 2000, MSD Animal Health initiated extensive epidemiological surveys in the major tilapia-producing regions of Asia and Latin America. It identified more than 1,000 bacterial isolates from tilapia reared at 74 sites in 14 countries to better understand the relative importance of streptococcal pathogens to the industry.
As other investigations have found, these streptococcal species were the dominant bacterial pathogens, accounting for more than half of all bacteria identified. However, it is interesting that while S. iniae was the most commonly reported pathogen of fish, the data showed that S. agalactiae was more prevalent in tilapia.
Biotype prevalence
Detailed analysis of the isolates revealed two distinct clusters that differed in a variety of biochemical and phenotypic characteristics. These clusters or biotypes typically differentiate between beta-haemolytical “classical” S. agalactiae (biotype I) and non-beta-haemolytical S. agalactiae (biotype II).
S. agalactiae biotype II was considered the more globally significant of the biotypes, with chronic mortality in many Asian and Latin American countries, whereas S. agalactiae biotype I was limited to Asia and displayed acute mortality peaks, often associated with higher temperatures.
In the authors’ epidemiological sur-veys to date, 26 percent of all streptococcal isolates of tilapia were found to be S. agalactiae biotype I, and 56 percent were identified as S. agalactiae biotype II. S. iniae made up 18 percent.

Vaccine development
Vaccines to protect against S. agalactiae have been described by various authors, but it is difficult to conclude from these studies if the vaccine and challenge strains were from the same or different biotypes.
To determine if S. agalactiae has consequences for the development of vaccines to control strep diseases, the authors conducted a laboratory challenge to determine the ability of biotype-specific vaccines to protect against lethal challenge with S. agalactiae biotype I or biotype II strains.
Tilapia vaccinated with experimental S. agalactiae biotype I vaccines were protected against S. agalactiae biotype I strains. However, no protection was
observed in tilapia that received the biotype I vaccine when they were challenged with virulent biotype II strains. Similarily, fish vaccinated with S. agalactiae biotype II vaccines were protected against lethal challenge with biotype II, but not biotype I strains. Thus, vaccination with biotype-specific bacterin vaccines induces biotype-specific protection against mortality caused by S. agalactiae.
Laboratory studies also demonstrated that AquaVac® Strep Sa, a biotype II vaccine ultimately developed for commercial use, protected against S. agalactiae biotype II for at least 30 weeks (Fig. 1).
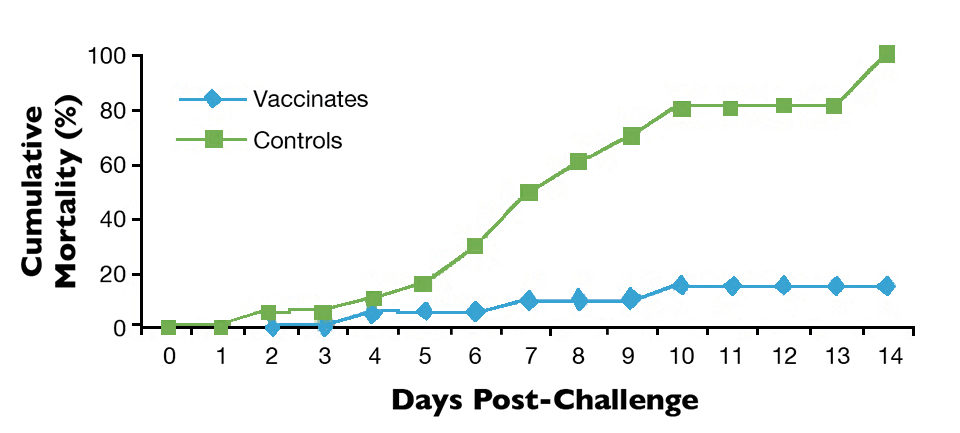
Field assessment
Carefully controlled field registration trials were conducted to determine if the results found in the lab for the prototype biotype II vaccine would be the same in the field.
The field trials were conducted in a lake environment using square cages housing about 10,000 fish at a large Asian tilapia farm. The trial was run in triplicate with three test cohorts including the biotype II vaccine, a placebo oil group and a negative, unvaccinated control. When the fish reached about 15 grams in weight, they were vaccinated after transfer to grow-out cages from the nursery ponds.
Extensive bacteriological and virological samplings were conducted before, during and every month after vaccination and at specific time points during the trial when mortality was recorded above “normal.” As per the routine growout strategy, the trial was terminated and fish were processed after about 200 days, when they reached approxmately 1.2 kg. Mortality, feed and water quality parameters were recorded daily along with the final harvest data.
Clear disease patterns emerged during the trial in all cages, indicating that columnaris disease or Flavobacterium columnare was responsible for high initial mortality peaks immediately after stocking. As the columnaris or “saddle-back” disease subsided, along with the initial transport and stocking stress, then irido-virus (a common viral disease of tilapia in the area) resulted in three clear peaks of mortality. This mortality pattern is often indicative of the disease and losses can routinely be as high as 30 percent.
As the fish got larger, S. agalactiae and, to a much lesser degree, S. inae were increasingly reisolated from moribund fish in all three cohorts. The mortality
patterns indicated that although the incidence of columnaris and iridovirus was similar in all groups, the degree of mortality from S. agalactiae was relatively much lower in the vaccine group when compared to the placebo oil and negative controls. Data gathered at harvest substantiated that observation: Survival in vaccinates was 80 percent compared to 67 percent survival in the placebo and negative-control groups, representing a 13 percent improvement in vaccinated fish.
Correspondingly, feed-conversion ratios (FCRs) were about 1.86 in the vaccinates, compared to 2.06 and 2.05 in the placebo and negative-control groups, respectively. This was an approximate 10 percent improvement in FCR (Table 1).
Wendover, Results from a field trial, Table 1
Treatment Group | Survival (%) | Feed-Conversion Ratio |
---|---|---|
Vaccinates | 80 | 1.86 |
Placebo-vaccinated controls | 67 | 2.06 |
Untreated controls | 67 | 2.05 |
In this trial, vaccinated fish had improved feed intake and feed utilization. Consequently, they had improved production efficiency performance, with 2.25 metric tons (MT) more fish harvested in the vaccinated population.
Further laboratory challenge studies using Indonesian, Malaysian, Vietnamese, Honduran, Brazilian, Mexican and Ecuadorian strains against the relative commercial vaccine indicated the vaccine cross-protects against multiple geographically diverse isolates.
Lessons learned
The success of the first large commercial-scale injection vaccination programs in tilapia to date has been variable, as expected, due to the presence of other diseases and other variables. The initial field trials and large-scale vaccination programs led to the identification of some fundamental factors to implement before any operation should embark on a vaccination program.
Correct diagnosis. Before a commercial producer commits to a vaccination program, the underlying cause of mortality needs to be determined by a fish health specialist.
Antimicrobial susceptibility analysis. If a bacterial disease is confirmed, a diagnostic laboratory should perform an antimicrobial susceptibility test to determine if the bacteria are susceptible to therapeutic remediation and, if this is the case, which antibiotic is most suitable to control the outbreak.
S. agalactiae biotype confirmation. If Streptococcus agalactiae is confirmed as the primary disease, a more detailed biotype analysis is necessary to ensure the right biotype-specific vaccine is chosen for the right reason.
Healthy fish. Healthy fish means that there is no sign of clinical or subclinical disease. Fish should be free of disease and stress before, during and for the first two to three weeks after vaccination. That means climatic (water quality) and housing conditions (biomass, handling, etc.) at this stage are crucial.
The best results are obtained in operations where hatchery, nursery, pre-growout and growout areas are separated from each other as well as housing units and equipment. The hatchery and nursery phases should be biosecure, well-controlled and disease-free. This not only gives juvenile fish the best start in life, but also allows vaccines to be administered in a controlled environment.
Components of vaccine program
There are four key components to effective vaccine programs.
Correct administration. The aim is to administer the vaccine in a consistent and proper manner so there is minimal stress on the animals. The correct dose must be given to each and every individual.
Sufficient immune response. Correct vaccination and maintenance in a healthy state under suitable housing conditions prior to transfer will trigger a sufficient immune response before exposure to the “challenge environment.”
Population protection. To achieve population protection, it is important that the entire population is vaccinated, not just a fraction of that population. The aim is to achieve blanket protection in the shortest time frame possible. This is much easier in an all-in/all-out production system, where 100 percent of stocked fish can be vaccinated.
Shift the balance. It is important to note that even if a fish has been vaccinated, it may still be susceptible to disease and infection depending on its health, nutritional and stress status. Therefore, a combination of good management, biosecurity, housing, water quality, nutrition, sanitation, immune stimulation and vaccination will tip the balance toward disease control.
Effective vaccination programs result in a rapid onset and full duration of protection. Over time, such programs have a self-perpetuating or “snowball” effect – with fewer sick and dying fish, there is less bacterial shedding in the water. Less bacterial shedding, in turn, lowers total challenge pressure. Coupled with increasingly more protected individuals, overall population performance will improve.
(Editor’s Note: This article was originally published in the September/October 2011 print edition of the Global Aquaculture Advocate.)
Authors
-
Neil Wendover, B.S.
Global Aquatic Animal Health
MSD Animal Health
1 Perahu Road
Singapore 718847[109,111,99,46,107,99,114,101,109,64,114,101,118,111,100,110,101,119,46,108,105,101,110]
-
Mario Aguirre
MSD Animal Health
1 Perahu Road
Singapore 718847 -
Rodrigo Zanolo, M.V., M.S.
MSD Animal Health
1 Perahu Road
Singapore 718847 -
Leonardo Cericarto, Ph.D.
MSD Animal Health
1 Perahu Road
Singapore 718847 -
Robin Wardle
MSD Animal Health
1 Perahu Road
Singapore 718847
Tagged With
Related Posts

Aquafeeds
A look at corn distillers dried grains with solubles
Corn distillers dried grains with solubles are an economical source of energy, protein and digestible phosphorus to reduce feed costs and fishmeal usage.

Health & Welfare
A study of Zoea-2 Syndrome in hatcheries in India, part 2
Indian shrimp hatcheries have experienced larval mortality in the zoea-2 stage, with molt deterioration and resulting in heavy mortality. Authors considered biotic and abiotic factors. Part 2 describes results of their study.

Health & Welfare
Bacillus probiotics benefit tilapia rearing under challenging conditions in Brazil
A recent study that evaluated the benefits of using probiotics with a balanced mixture of Bacillus bacteria strains to inhibit pathogenic bacteria in tilapia.

Health & Welfare
Developing live bacterial vaccines by selecting resistance to antibacterials
As wide use of antibiotics has led to antibiotic resistance in fish pathogens, vaccines present an alternative control method to prevent bacterial diseases.