Hydrogen sulfide produced in anoxic zones can be toxic
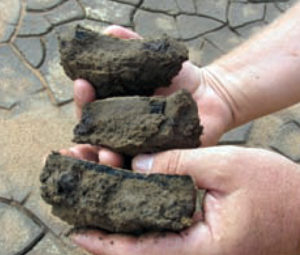
Sulfur is an essential nutrient for living things. Although it has several roles in nutrition, it is most important as a component of certain amino acids. Sulfate is the main source of sulfur for most plants. Plants use the compound to synthesize sulfur-containing amino acids that are passed on to animals via the food web.
When plants and animals die, their remains are decomposed by bacteria and other microorganisms that also require sulfur. Organic residues usually contain more sulfur than needed by decomposers, and sulfur is released to the environment as sulfide. In the presence of molecular oxygen, sulfide is quickly oxidized to sulfate. Conversely, in the absence of molecular oxygen, sulfate is reduced to sulfide.
Sulfur cycle
Almost everyone has heard of the global carbon and nitrogen cycles, but the global sulfur cycle (Fig. 1) is less familiar. Because carbon, nitrogen, and sulfur are components of living organisms, their cycles obviously are intertwined. Also, like the other global cycles, many of the aspects of the sulfur cycle operate in aquaculture ponds.
Sulfur is not as important as nitrogen and phosphorus as a nutrient-limiting factor for aquatic plant growth. This is especially true in marine and estuarine aquaculture – normal seawater contains 2,700 milligrams per liter of sulfate. Sulfur, nevertheless, can be a major factor in aquaculture. Ponds constructed in areas where soils contain ferrous sulfide can have severe problems with low pH in culture water. In addition, hydrogen sulfide produced in anoxic zones of ponds can be toxic to aquatic animals.

Pyritic problems
In mangrove forests and other coastal wetlands, plants trap sediment and their remains add organic matter to it. Soil particles in sediment contain ferric iron compounds, and the surrounding water is high in sulfate concentration. High rates of microbial degradation of organic matter can cause anaerobic zones in sediment. Ferric iron on soil particles is reduced to soluble ferrous iron, and sulfate contained in sediment pore water is reduced to sulfide. Ferrous iron and sulfide react to form insoluble iron pyrite, which precipitates in the sediment.
Iron pyrite is stable under anaerobic conditions and can accumulate over time. Total sulfur concentrations in some coastal soils can reach 5 or 6 percent. Sedimentation can eventually lead to the formation of new land along the edges of coastal marshes with a layer of pyritic material 50 to 100 cm below the surface.
Construction of ponds in coastal areas can expose pyritic soil in bottoms and on embankments. Exposed iron pyrite oxidizes with release of sulfuric acid. The oxidation rate of iron pyrite is greatly accelerated by the activity of several species of bacteria of the genus Thiobacillus. These chemoautotrophic organisms are capable of oxidizing sulfide to sulfate and using some of the resulting energy to synthesize organic matter by a non-photosynthetic pathway.
PAS soil
Before being exposed to the air, pyritic soils are called potential acid-sulfate (PAS) soils. Such soils can have pH values of 5 to 7 while wet. Upon drying and exposure to the air for a few days, the pH of PAS soils usually drops to 2 or 3. The soils then are known as acid-sulfate soils. Some PAS soils contain carbonate that neutralizes the acidity to prevent the pH decline, but this is rare.
Acidity produced by pyrite oxidation neutralizes total alkalinity and causes the pH of pond waters to decline, potentially to a dangerous level. For example, I am aware of fish ponds in the Philippines that were unknowingly constructed in PAS soil. When the rainy season began, acidity leached from embankments into the ponds and the fish died from low pH. Low pH caused by pyrite oxidation can also dissolve aluminum and other metals that are harmful to aquaculture species.
In anaerobic sediment and water, certain heterotrophic bacteria respire using oxygen in sulfate as a substitute for molecular oxygen. Electrons and hydrogen ions released by the bacterial respiration combine with oxygen in sulfate and produce sulfide. pH and temperature regulate the proportions of hydrogen sulfide, hydrosulfide anion, and sulfide.
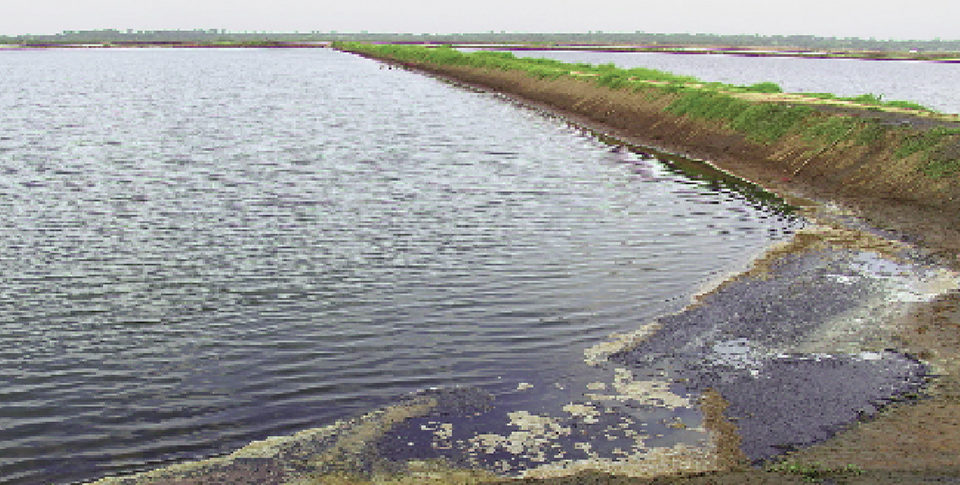
Hydrogen sulfide
Hydrogen sulfide is extremely toxic to fish, shrimp, and other aquatic organisms. Shrimp and other crustaceans possibly are more tolerant to hydrogen sulfide than fish, but few data are available to confirm this. The ionic forms of sulfide are not as toxic as hydrogen sulfide, whose concentration can be estimated for the appropriate pH and water temperature (Table 1).
Boyd, Hydrogen sulfide as a percentage of total sulfide in water, Table 1
pH | Temperature (° C) 16 | Temperature (° C) 20 | Temperature (° C) 24 | Temperature (° C) 28 | Temperature (° C) 32 |
---|---|---|---|---|---|
5.0 | 99.3% | 99.2% | 99.1% | 98.9% | 98.9% |
5.5 | 97.7% | 97.4% | 97.1% | 96.7% | 96.3% |
6.0 | 93.2% | 92.3% | 91.4% | 90.3% | 89.1% |
6.5 | 81.2% | 79.2% | 77.0% | 74.6% | 72.1% |
7.0 | 57.7% | 54.6% | 51.4% | 48.2% | 45.0% |
7.5 | 30.1% | 27.5% | 25.0% | 22.7% | 20.6% |
8.0 | 12.0% | 10.7% | 9.6% | 8.5% | 7.6% |
8.5 | 4.1% | 3.7% | 3.2% | 2.9% | 2.5% |
9.0 | 1.3% | 1.2% | 1.0% | 0.9% | 0.8% |
Aquaculture ponds usually do not stratify thermally, and anaerobic conditions are restricted to their sediments. Hydrogen sulfide produced in sediment usually does not enter the water column if an aerobic layer occurs at the sediment-water interface, for sulfide entering this layer would be oxidized. The absence of an aerobic layer at the sediment-water interface favors movement of sulfide into the water column, where it is oxidized to sulfate. However, if sulfide enters the water column faster than it can be oxidized, a residual and possibly toxic concentration of sulfide can result.
Soil evaluations for aquaculture projects should include tests for PAS soils. The most definitive test is to measure the total soil sulfur concentration. Sulfur concentrations above 0.75 percent indicate PAS conditions. Another test for PAS soil is to dry a sample and measure whether its pH drops drastically. The pH can fall below 4.0 in a strongly pyritic sample.
Suggested pond practices
Production of acidity is usually not great as long as PAS soils are submerged or not exposed to air. It often is possible during construction to cover pyritic soil with a layer of nonpyritic material. Embankments also should be planted with grass, as this reduces exposure to air.
Agricultural limestone should be applied to ponds to raise alkalinity above 75 milligrams per liter. The soil on embankments also should be treated at 0.2 to 0.3 kilograms per square meter with agricultural limestone to improve conditions for grass.
When ponds are drained for harvest and dried, they should be partially refilled and the water discharged to flush out acidity. Before refilling, agricultural limestone should be spread over the bottom at 2,000 to 3,000 kilograms per hectare. Limestone applications must be applied after each crop, for pyritic soils can generate acidity for years. The problem, however, should decrease over time in response to management.
High concentrations of hydrogen sulfide are more likely in brackish water or seawater ponds because their waters contain more sulfate than freshwater systems. The best approach to avoiding hydrogen sulfide problems in aquaculture ponds is attention to good pond bottom soil and water quality management.
Pond bottoms should be dried out between crops, excessive sediment removed, and lime applied if pH is below 7.5. Good feed management should be used to prevent overfeeding, accumulation of uneaten feed, and resulting anoxic conditions in bottom areas. Mechanical aeration to prevent thermal stratification and move oxygenated water over pond bottoms also is important.
Application of hydrated or burnt lime to quickly increase pH to 8.5 or 9.0 will reduce the proportion of hydrogen sulfide when total sulfide concentration is high. Liming can be an effective emergency measure for preventing sulfide toxicity.
(Editor’s Note: This article was originally published in the November/December 2007 print edition of the Global Aquaculture Advocate.)
Now that you've finished reading the article ...
… we hope you’ll consider supporting our mission to document the evolution of the global aquaculture industry and share our vast network of contributors’ expansive knowledge every week.
By becoming a Global Seafood Alliance member, you’re ensuring that all of the pre-competitive work we do through member benefits, resources and events can continue. Individual membership costs just $50 a year. GSA individual and corporate members receive complimentary access to a series of GOAL virtual events beginning in April. Join now.
Not a GSA member? Join us.
Author
-
Claude E. Boyd, Ph.D.
Department of Fisheries and Allied Aquacultures
Auburn University
Auburn, Alabama 36849 USA[117,100,101,46,110,114,117,98,117,97,64,49,101,99,100,121,111,98]
Tagged With
Related Posts

Responsibility
Hydrogen sulfide toxic, but manageable
Hydrogen sulfide, which can form in pond bottom sediment, is toxic to aquatic animals because it interferes with reoxidation of cytochrome a3 in respiration.

Responsibility
A review of water quality improvement products
Prof. Boyd examines products used by aquafarmers to improve water quality and conditions in their ponds and discusses their efficacy.

Health & Welfare
Beneficial microbes and pathogen control
A range of alternative products is available to improve animal health and water quality, and control pathogen loads. Beneficial microbes produce antimicrobial compounds and suppress pathogen proliferation.

Responsibility
Assessing groundwater quality in aquaculture
Those interested in using groundwater for aquaculture should perform a thorough chemical analysis of the water. Several problems related to groundwater use in hatcheries and holding or transport vessels can be alleviated by degassing or aeration.