Pace of development may narrow the gap between aquaculture and crop and livestock breeding
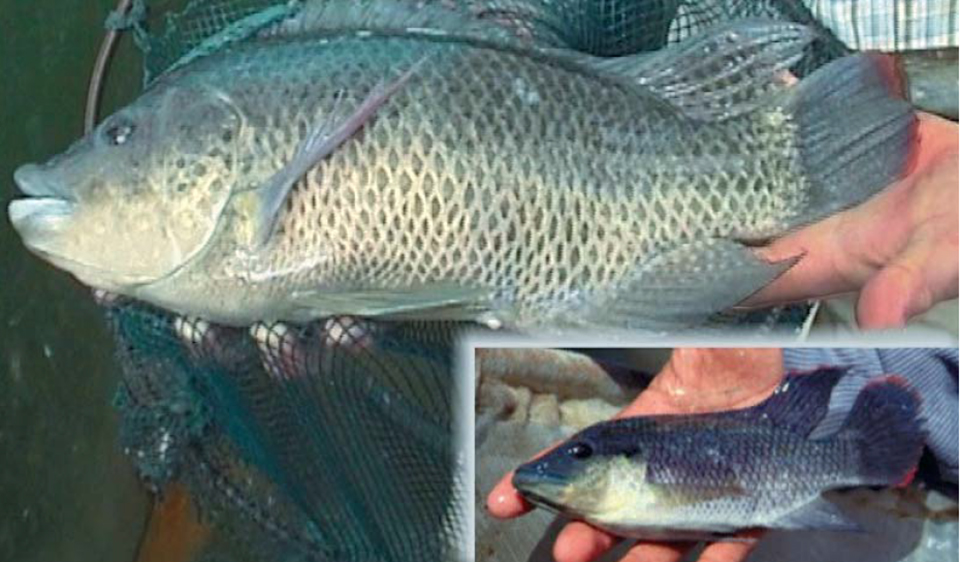
poor culture potential of the Asian O. mossambicus (inset) compared to wild-caught stocks in southeast Africa.
Based on regular contact with tilapia farmers over the past 15 years, it is apparent that the genetic quality of tilapia used in aquaculture is usually a minor concern and is often ignored completely. Even in largescale commercial operations or stateof- the-art intensive-production systems, due consideration is rarely given to the origin or quality of the stock cultured other than ensuring the correct species is used.
Tilapia species
Maternal mouthbrooding tilapia from the genus (Oreochromis) dominate aquaculture. Throughout much of the world – particularly in Asia, where more than 80 percent of cultured tilapia is produced – the black tilapia (O. mossambicus) was the first to be introduced, becoming widespread in the 1960s. Relatively poor culture performance and a tendency to stunting led this species to be largely displaced in most aquaculture systems (although large feral populations still exist) by (O. niloticus).
Best species
It is commonly considered that O. niloticus is the best tilapia species for tropical freshwater culture, while O. mossambicus and its hybrids have benefits in brackish water. O. aureus, or more often its hybrid with O. niloticus, is grown in some regions, due in part to its cold tolerance.
Genetic bottlenecks
Commonly, new introductions of tilapia take place with relatively small numbers of fish leading to strong founder effects. For example, it is thought that virtually all the O. mossambicus in Asia are derived from five individual fish discovered in Indonesia in the 1930s.
This represents a very significant genetic bottleneck, and explains the very low levels of genetic diversity of Asian O. mossambicus compared to indigenous populations from southeast Africa (Fig. 1).
Due to its popularity, there have been more frequent introductions of Nile tilapia into aquaculture from wild African populations. As a result, this species appears to be more robust and less prone to problems associated with founder effects.
Isolated populations
Due to their ease of breeding, tilapia are often maintained as reproductively isolated populations, with the result that inbreeding, genetic drift (random genetic changes), and unconscious selection can impact their genotypic and phenotypic properties. Poor broodstock management practices – such as mating of relatives and unconscious selection – commonly lead to a deterioration in the genetic quality of domesticated populations. Many such populations are inferior to wild-caught stocks, even though the latter are often not particularly well adapted to the captive environment.
Do strains exist?
In animal and plant breeding, the term “strain” is normally applied to intraspecific subpopulations that exhibit distinctive traits, which are normally homozygous or true breeding. However, in fish and particularly in tilapia, strains or isolates are normally loosely designated according to their location or origin, and commonly have no distinctive traits.
There are numerous so-called “strains” of tilapia throughout the world, and several are promoted as having particularly desirable traits for aquaculture. However, in reality, few of these strains are sufficiently distinctive to truly warrant the name. Apart from the obvious example of the red tilapia, most tilapia producers would be hard pressed to discriminate one strain from another.
Growth rates
Despite the fact that tilapia strains are not easily distinguished by their appearance or properties, several scientific studies have demonstrated that there can be significant differences between strains for commercially important traits, with differences in growth rates of up to 20 percent as an example. Furthermore, genotype/environment interactions, in which the relative performance of strains differ between environments, are common.
It is thus often worthwhile for producers to evaluate available strains to identify those best suited to their systems (Table 1). Differences in growth rates of a few percent or a 1 percent increase in fillet yield can have significant impacts on the profitability of tilapia culture, especially in markets where profit margins are slim.
Mair, Summary of genetic technologies, Table 1
Technology | Species | Benefit | Uptake |
---|---|---|---|
Selective Breeding | O. niloticus (GIFT) | Up to 85% improvement in growth rates compared to base population | Introduced for aquaculture in several Asian countries with expanding adoption. |
Hybridization | Mainly F1 O. niloticus x O. aureus hybrids and O. mossambicus- based red tilapia | Can produce high percentage of male progeny and extend environmental tolerances. | O. n x O. a hybrid produced widely in some areas, particularly China. O. mossambicus-based hybrids (particularly red tilapia) cultured in brackish water. |
Sex Control | O. niloticus | YY male technology produced high-yielding monosex genetically male tilapia. | Now cultured in over 25 countries. |
Transgenesis | O. u. hornorum-based hybrid | Significant increases in growth. | Thought to be now cultured in Cuba, where it was developed. Unlikely to be approved for culture elsewhere in the near future. |
Selective breeding
Several research projects have evaluated selective breeding for various traits in tilapia. Response to selection has been demonstrated for such traits as age at maturation and cold tolerance, although the major emphasis of most studies is on growth-related traits. Commercial-scale selection programs for increased growth rate have been successfully carried out over several generations.
GIFT project
Probably the best-known example of selection for growth is the ICLARM-coordinated Genetic Improvement of Farmed Tilapia project. This project, based in the Philippines, used a combined selection methodology on a synthetic base population developed from newly introduced stocks from Africa and domesticated Asian stocks. The program achieved genetic gains averaging 13 percent over five generations, providing an estimated cumulative increase of 85 percent in growth rate compared to the base population.
While these genetic gains are significant and clearly demonstrate the benefits of well-organized breeding programs, the accumulated response to selection appears not to be fully expressed in all culture environments. Difficulties in identifying adequate controls have created problems in the accurate assessment of genetic gains.
Molecular genetics
Recent advances in molecular genetics are likely to provide tools that can enhance traditional selectivebreeding methods. Gene mapping can be used to identify genes directly or indirectly linked to the expression of specific traits known as quantitative trait loci. Identification of these traits, particularly those difficult to quantify based only on phenotype (fillet yield, disease resistance, environmental tolerance, etc.) could enable selection for or against these traits in marker-assisted selection programs that are likely to enhance the benefits of traditional selection.
Hybridization and crossbreeding
Numerous studies on hybridization between species have resulted in more than 60 different hybrids between and among the Oreochromis and Sarotherodon tilapia, with the majority between Oreochromis species. However, there have been few studies on crossbreeding within species strains.
Hybrid vigor
The main objective of such studies is to identify heterosis or “hybrid vigor” for commercially important traits. Positive heterosis occurs when the value of a trait in a hybrid or crossbred is greater than that of the parent species/strains. Virtually all reports of hybridization show that hybrids within and between the tilapia genera are viable, indicating the speciation within the tilapine fishes may be relatively recent.
Furthermore, among the tilapia hybrids, there are no reports of sterility commonly found in hybrids of other species groups. Despite many hybridizations, there are few, if any, published studies that clearly demonstrate heterosis for any commercially important trait, with values for such traits in hybrids commonly falling between those of the parental species.
Male surplus
Oreochromis hybrids are characterized by a surplus of males. The occurrence of all-male broods is not uncommon, and this is where the major interest in hybridization lies. Table 2 summarizes the hybrid combinations known to produce monosex male progeny.
Mair, Summary of hybrid combinations, Table 2
Female Parent | Male Parent | Note |
---|---|---|
O. niloticus | O. aureus | Applied commercially but results inconsistent. |
O. niloticus | O. macrochir | |
O. niloticus | O. urolepis hornorum | Majority of broods are all-male. Some commercial application. |
O. niloticus | O. variablis | All progeny were monosex. |
O. mossambicus | O. aureus | |
O. mossambicus | O. urolepis hornorum | All progeny were monosex. |
O. spilurus niger | O. macrochir | |
O. spilurus niger | O. urolepis hornorum | All progeny were monosex. |
O. aureus | O. urolepis hornorum | |
T. zillii | O. andersonii | All progeny were monosex. |
Attempts to commercialize monosex hybrids, usually with the O. niloticus × O. aureus cross or using male O. urolepis hornorum with females from O. niloticus or O. mossambicus, have met with varied success. Hybrids involving O. hornorum are not widely cultured, despite the consistency of monosex sex ratios, due to the relatively poor growth performance of the species.
The O. niloticus × O. aureus hybrid often produces inconsistent sex ratios, and broodstock contamination can lead to further deviations from predicted sex ratios. However, this hybrid is thought to dominate tilapia production in China, which produces more than half the world’s cultured tilapia. It is not known what sex ratios are obtained in these mass-produced hybrids. Amajor factor behind this production may be the greater cold tolerance of this hybrid than that of the faster-growing pure O. niloticus.
Crossbreds
The few studies that looked at crossbreds (i.e., crosses between stocks, within species) have not demonstrated significant heterosis, although crossbreds have been produced to provide a more genetically variable base population for selective-breeding programs.
Sex control
With the failure of hybridization to effectively solve the problem of early sexual maturation, unwanted reproduction, and overpopulation in tilapia culture, alternative technologies were sought. One popular alternative is hormonal sex reversal, but this technique has a number of technical, environmental, and social constraints. Thus, an alternative genetics-based solution has been sought, founded on the current knowledge of the mechanisms of inheritance of sex in tilapia.

YY “supermales”
Based on the theory of predominantly monofactorial sex determination in the commercially important Nile tilapia O. niloticus, it has proved possible to manipulate sex ratio using a combination of sex reversal and progeny testing to identify sex genotypes. In a major breeding program in this species, it has proved feasible to mass-produce novel YY males. When crossed to normal females (XX), these YY “supermales” have the unique property of siring only genetically male progeny.
Genetically male tilapia
Termed genetically male tilapia, these progeny are normal (XY) genetic males with an average sex ratio of over 95 percent male. They are not classed as genetically modified organisms, and the technology can be applied in a range of hatchery systems simply by replacing broodfish with YY males, although good broodstock management is required to prevent contamination. Growth trials have produced enhanced yields 30-90 percent higher than equivalent mixedsex tilapia.
The YY male technology has now been combined with other genetics-based breeding methods to further enhance yields. Recent trials of genetically male tilapia produced using a selected female line have produced further gains in growth rate. A similar, although slightly simpler, breeding program has been developed to produce monosex male O. aureus, through the production of sex-reversed ZZ females, although this monosex fish is not thought to be in significant commercial production.
Genetic manipulations
Two types of genetic manipulations have been successfully carried out in tilapia: chromosome set manipulations, including gynogenesis, androgenesis, and triploidy; and gene transfers to produce transgenic lines.
Chromosome set manipulations
There has been a significant and largely successful research effort on chromosome set manipulations in several tilapia species. Inbred uniparental lines of maternal and paternal origins have been produced by gynogenesis and androgenesis, respectively. Mitotic gynogenesis and androgenesis have also been used to produce isogenic clonal lines.
These techniques have provided useful research tools – in the elucidation of sex determination, for example – but have not produced outputs of great commercial interest. The main commercial interest in chromosome set manipulation is in the production of sterile triploids. These can be produced by application of physical shocks (heat, cold, or pressure) to eggs shortly after fertilization.
Triploids have comparable or slightly slower growth rates than normal diploids, but offer the significant advantage of sterility. However, triploids can only be produced using artificial fertilization, which is not possible on a commercial scale. Attempts to produce viable tetraploids that could then be used to mass-produce triploids in crosses with normal diploid fish have, to date, not met with success.
Gene transfer
Transgenesis is one of the most promising technologies for generating relatively rapid genetic improvements. It involves the introduction of exogenous genes into eggs at early stages of development to confer novel phenotypic characteristics. Two major published programs have produced truebreeding, enhanced transgenic lines.
A program in Cuba has produced a true-breeding line, transgenic for a tilapia growth hormone gene, in an O. u. hornorum-based hybrid tilapia. These transgenic fish have been shown to grow 55 percent faster than nontransgenic fish. It is thought this fish has been approved for aquaculture in Cuba and is now under production.
A program based in the United Kingdom has produced a line of O. niloticus that is transgenic for a salmon growth hormone gene. The expression of this transgene resulted in dramatic growth enhancement. Average weight of the transgenic fish was three to four times that of their nontransgenic siblings (Fig 2), with equivalent or superior food-conversion efficiencies.
Transgenesis: potential vs. risk
While the potential of transgenesis is evident, the rate of genetic change in transgenic organisms is such that their phenotypic and behavioral properties cannot easily be predicted. The introduction of these transgenic fish for commercial aquaculture therefore faces many constraints.
The risks to the environment posed by the uncontrolled introduction of transgenic fish needs to be adequately assessed prior to their adoption for aquaculture. Many concerns could be effectively addressed if guaranteed sterile transgenic tilapia can be produced. Attempts are already under way to develop a selectively sterile fish using transgenesis.
Consumer response to transgenic fish, which are classed as genetically modified organisms, is also generally negative. Particularly in developed countries, adoption of transgenics by farmers may involve significant economic risks.
Conculsion
Today, with the application of genetics in aquaculture still in its embryonic stages, more careful choices of strains and improvements to basic broodstock management practices can bring significant increases in returns to commercial tilapia producers. The ease of handling and domestication of tilapia, together with their reproductive characteristics and relatively short generation time, make them an ideal species for further research. Although aquaculture genetics lag behind advances in crop and livestock breeding, the current pace of development may narrow the gap appreciably in coming years.
(Editor’s Note: This article was originally published in the December 2001 print edition of the Global Aquaculture Advocate.)
Now that you've finished reading the article ...
… we hope you’ll consider supporting our mission to document the evolution of the global aquaculture industry and share our vast network of contributors’ expansive knowledge every week.
By becoming a Global Seafood Alliance member, you’re ensuring that all of the pre-competitive work we do through member benefits, resources and events can continue. Individual membership costs just $50 a year. GSA individual and corporate members receive complimentary access to a series of GOAL virtual events beginning in April. Join now.
Not a GSA member? Join us.
Author
-
Graham C. Mair, Ph.D.
School of Biological Sciences
University of Wales Swansea, U.K.
Asian Institute of Technology
P.O. Box 4
Klong Luang, Pathumthani 12120
Thailand[104,116,46,99,97,46,116,105,97,64,114,105,97,109,99,103]
Tagged With
Related Posts

Health & Welfare
Bath immersion, booster vaccination strategy holds potential for protecting juvenile tilapia
In a study, vaccination of juvenile tilapia by bath immersion followed by two booster vaccinations stimulated specific antibody responses and protection against S. iniae challenge.

Responsibility
‘Model’ tilapia venture shows mettle in Mozambique
On the shores of Lake Cahora Bassa, Chicoa Fish Farm hopes to create a ripple effect to improve fish supply and quality of life for an impoverished region.

Health & Welfare
Domestication and broodstock management
A domesticated organism is trained to live with, or be of service to, humans. In practice, their life cycle has been closed in artificial environments.

Health & Welfare
Effect of dietary salt on Nile tilapia
Study examines impact of an increase in dietary NaCl on nutritional and other physiological processes, and effects on gut microbiome in Nile tilapia.