Use of oxygen gas can be conserved by using a continuous oxygen-monitoring system capable of controlling oxygen injection
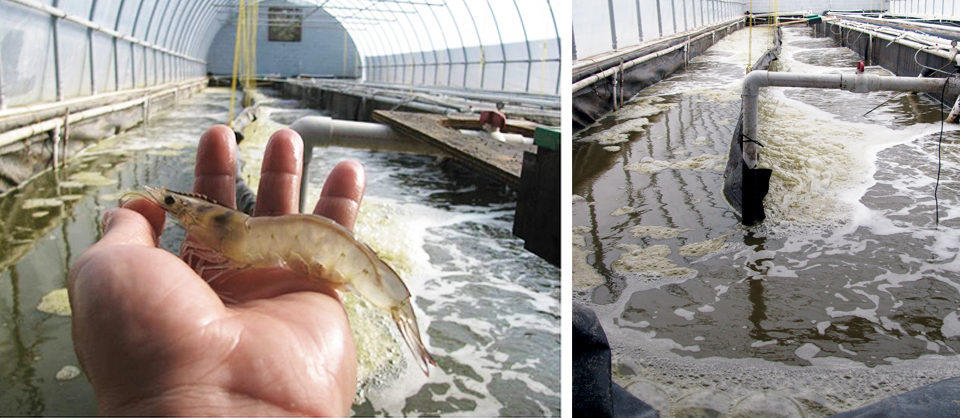
Intensive biofloc shrimp culture systems allow for indoor production, low water-exchange rates and high biosecurity levels. Management of these systems varies, but one strategy is to add labile organic carbon sources to the water to raise the carbon:nitrogen ratio and facilitate heterotrophic assimilation of otherwise toxic nitrogen compounds.
Heterotrophic microbes in the water use carbon for energy and build proteins from nitrogen. These microbes may then provide supplemental nutrition for shrimp. This technique has the potential to increase shrimp growth rates and lower feed-conversion ratios (FCRs).
The authors conducted a trial to evaluate the use of a heterotrophic biofloc system equipped with the latest in aeration and centralized heating technology to achieve good shrimp production.
Raceway setup
A 50-m3 concrete raceway measuring 30.1 m long, 3.2 m wide and 0.5 m deep was lined with high-density polyethylene plastic and contained under a greenhouse covered in clear plastic. Water was propelled around a central wall using two 1.5-hp pumps. Each pump was connected to seven nozzles evenly spaced near the bottom of one length of the raceway. The nozzles drew air through a 2.5-cm-diameter pipe that extended above the water surface. One such pipe was outfitted to receive pure oxygen gas, which was injected on a precautionary basis at a rate of approximately 2 L/minute.
The project was conducted during winter months and therefore required supplemental heating. Two hot water boilers heated clean, fresh water that was moved through a central piping system via a pump. A digital thermostat maintained water temperature in the raceway at 29 degrees C. The thermostat operated a secondary pump that, when needed, moved hot water through a titanium heat exchanger within the raceway.
Just outside the greenhouse was a 760-L settling chamber with a cone-shaped bottom and a 10-cm-diameter pipe suspended in the center. Water from the raceway was continuously pumped through the settling chamber at a rate of approximately 15 L/minute. Solids settled at the bottom and were removed weekly, while clarified water near the top flowed back to the raceway.
Shrimp culture
Shrimp were grown in a nearby nursery raceway until they weighed an average of 1.5 g, at which point they were stocked into the experimental production raceway at a density of 250 shrimp/m3. The shrimp were originally fed rations based on an assumed FCR of 1.5:1, growth of 1.8 g/week and assumed mortality rate of 8 percent at stocking and 1 percent/week thereafter. However, this feeding rate was adjusted by routinely sampling for uneaten feed using a dip net.
Feed rations were set such that no uneaten feed could be found approximately 30 minutes prior to each subsequent feeding, and no feed could be found prior to the first feeding of the day. Seventy percent of the daily feed ration was dispersed evenly throughout the raceway by hand, and the remaining 30 percent was placed on two 12-hour belt feeders for overnight feeding. Shrimp weights were sampled weekly, salinity was maintained at 20 ppt, and shrimp were grown for 82 days.
Granulated sucrose was added daily at a rate of 50 percent of the wet weight of added feed to raise the carbon:nitrogen ratio and encourage the function of heterotrophic microorganisms. In a few instances where dissolved nitrite concentration was high, extra sucrose was added to further increase nitrogen assimilation. The carbon and nitrogen contributions of the feed and sucrose are shown in Table 1.
Ray, Carbon and nitrogen contributions, Table 1
Carbon (%) | Nitrogen (%) | Weight (kg) | Carbon Added (kg) | Nitrogen Added (kg) | Carbon: Nitrogen Ratio | |
---|---|---|---|---|---|---|
Feed | 41.7 | 5.8 | 348.0 | 145.1 | 20.2 | 7.2 |
Sucrose | 41.0 | 0 | 179.6 | 73.7 | 0 | – |
Total | 82.7 | 5.8 | 527.6 | 218.8 | 20.2 | 10.8 |
Temperature, pH and dissolved-oxygen levels were measured twice daily, and other water quality parameters were measured weekly. Morning pH readings were used to decide how much sodium bicarbonate to add each day in an effort to maintain high pH during the project. If pH was below 7.9, 300 g sodium bicarbonate were added, and 500 g were added if pH fell below 7.7. For pH below 7.5, 1,000 g were added.
Results
Water temperatures did not fluctuate substantially between morning and afternoon measurements, likely because the air temperature remained low, and water temperature was influenced primarily by the heating system. High pH was maintained near 7.8 through regular sodium bicarbonate additions. Dissolved oxygen was held at a relatively high concentration by using the aeration nozzles and added oxygen gas. Mean values for these and other water quality parameters are given in Table 2.
Ray, Mean water quality parameters, Table 2
Parameter | Value |
---|---|
Temperature | 29.0 ± 0.5° C |
pH | 7.8 ± 0.2 |
Dissolved oxygen | 7.9 ± 0.8 mg/L |
Ammonia nitrogen | 0.2 ± 0.2 mg/L |
Nitrite nitrogen | 2.3 ± 1.9 mg/L |
Total suspended solids | 337.0 ± 52.0 mg/L |
Turbidity | 84 ± 15 NTU |
Settled solids | 14.5 ± 3.7 mL/L |
Ammonia concentrations remained low during the project, but nitrite concentrations as high as 5.5 mg/L were measured near the middle and end of the project. However, no mortality or decline in feeding behavior was observed in relation to the nitrite spikes. Although nitrate concentration was not measured, other raceways managed to favor heterotrophic nitrogen assimilation at the Gulf Coast Research Laboratory had very little nitrate accumulation.
Total suspended solids (TSS) concentrations were maintained slightly higher than what other authors have recommended for biofloc systems, but shrimp production metrics did not seem to reflect any adverse effects. Also, the management of solids concentration with a settling chamber resulted in a total water exchange of 5.8 percent of the volume of the raceway, not including water lost to evaporation. When the operating salinity of 20 ppt is mathematically adjusted to the 30-ppt levels in recent studies by other authors, the total water use would be 133 L/kg shrimp biomass. At 35 ppt, the salinity value of full-strength seawater, water use would have been 114 L/kg shrimp.
The average shrimp growth rate was 1.8 g/week. Shrimp grew from 1.5 to 22.5 g in 82 days, indicating that if the conditions of this project can be maintained, it may be possible to grow more than four crops of shrimp yearly. FCR was very low at 1.2:1, and survival was high at 96.6 percent. Although the stocking density was lower than in similar projects, the biomass production was relatively high at 5.3 kg/m3 due to high survival and moderate final weights of 22.5 g.
Perspectives
This raceway project represented an effort to combine some of the latest technological innovations with biofloc management regimes that have been in development at the Gulf Coast Research Laboratory. The consistent water temperature provided by the central heating system likely contributed to good shrimp production. Constantly high dissolved-oxygen (D.O.) concentrations probably assisted the production metrics as well, although a lower D.O. concentration may have been adequate.
The authors are confident that the use of oxygen gas can be conserved by using a continuous oxygen-monitoring system capable of controlling oxygen injection. The aeration system and water pumps may be capable of maintaining adequate D.O. concentration without supplemental oxygen.
Consistently high pH was accomplished using regular bicarbonate inputs. However, it is unclear how this may affect the mineral composition of the culture water if it is reused for multiple shrimp crops. Heterotrophic nitrogen assimilation functioned well, although spikes in nitrite were troubling. The process of assimilation may be facilitated more effectively using feeds with lower protein content.
The shrimp production parameters were very good, most notably growth rate, FCR and survival. These results, along with low rates of water use, may provide impetus for using this technology at inland locations, especially in cooler climates.
(Editor’s Note: This article was originally published in the January/February 2014 print edition of the Global Aquaculture Advocate.)
Authors
-
Andrew J. Ray, Ph.D.
Department of Coastal Sciences
Gulf Coast Research Laboratory
University of Southern Mississippi
703 East Beach Drive
Ocean Springs, Mississippi 39564 USA[109,111,99,46,108,105,97,109,103,64,121,97,114,106,119,101,114,100,110,97]
-
Jeffrey M. Lotz, Ph.D.
Department of Coastal Sciences
Gulf Coast Research Laboratory
University of Southern Mississippi
703 East Beach Drive
Ocean Springs, Mississippi 39564 USA
Tagged With
Related Posts

Aquafeeds
Biofloc and clear-water RAS systems: a comparison
This study compared two types of indoor, shrimp culture systems: clear-water RAS and biofloc systems. Clearwater RAS had the edge in water quality, but shrimp in the biofloc treatment had a higher feed conversion ratio.

Aquafeeds
Biofloc systems viable for tilapia production
Well-designed and managed biofloc technology systems are a viable alternative for production of various species like tilapia, to increase feed efficiency by lowering aquafeed protein requirements and to help reduce or eliminate effluent discharges.

Health & Welfare
Biofloc technology holds potential for carnivorous fish species
Juvenile carnivorous African catfish performed well in biofloc-based systems, which could help produce better quality and more disease-resistant seed of this important aquaculture species and support the expansion of African catfish farming industry.

Aquafeeds
A look at protease enzymes in crustacean nutrition
Food digestion involves digestive enzymes to break down polymeric macromolecules and facilitate nutrient absorption. Enzyme supplementation in aquafeeds is a major alternative to improve feed quality and nutrient digestibility, gut health, compensate digestive enzymes when needed, and may also improve immune responses.