Concepts, considerations and limitations
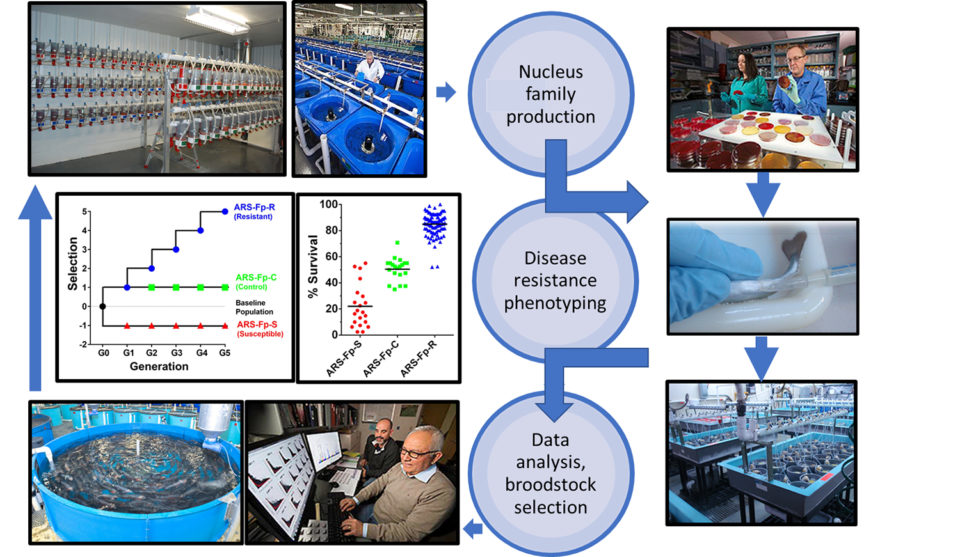
Selective breeding is the intentional selection and mating of parents with desirable traits. Selective breeding programs with well-defined breeding objectives have been responsible for dramatic improvements in terrestrial livestock production performance. For example, since about 1960 the average 56-day-old broiler weight has quadrupled, with 85-90 percent of the improvement due to genetic improvement, and Holstein milk yield has doubled.
Genetic improvement of farmed aquatic species is still in its infancy, but there is strong consensus of the potential to substantially improve performance for important production-related traits like growth, carcass composition and feed efficiency.
Disease resistance, a complicated trait
Genetic improvement of disease resistance traits in animal populations is more complicated compared to traditional production traits like growth because of the following reasons.
First, there currently is no easy or convenient method to measure host resistance; animals must be exposed to the pathogen and develop disease to accurately measure the resistance (or tolerance) phenotype. However, the need to maintain specific pathogen free animals in the nucleus population usually precludes the selection of broodstock that survived and recovered from a disease challenge. Thus, like other lethally-measured traits or sex-limited traits, disease resistance generally cannot be directly measured on breeding candidates.
Second, there is tremendous variation among the different bacterial, viral and parasitic microorganisms and the diseases they cause, and even considerable variation between pathogen isolates of the same species.
Third, a standardized, high-throughput, repeatable laboratory challenge model that mimics a natural disease challenge is important to accurately measure the disease resistance phenotype on all nucleus families; developing this model is not always trivial or possible under laboratory settings.
Fourth, there is currently a limited understanding of the trade-offs when selecting for disease resistance. Specifically, how does selection for specific disease resistance impact other production-related traits or resistance to other pathogens?
Finally, whereas there is a long history of selective breeding for disease resistance in plants, there are only limited examples in terrestrial species and thus uncertainty with respect to the potential for successful application.
Advantages of salmonids
Salmonid species have advantages compared to other terrestrial species with respect to selective breeding for disease resistance, including artificial spawning, which facilitates specific matings between individual males and females. Temperature-synchronized embryo development allows hundreds of families to be fertilized over a six-week period yet hatch within a five-day period.
Large full-sib family sizes (thousands of individuals) permit large-scale sib testing from which family-specific breeding values can be estimated and pathogen-naïve siblings can be selected for replacement broodstock based on family genetic merit. The high fecundity of salmonid species also allows for rapid amplification and dissemination of truly elite genetics. Finally, there generally seems to be sufficient genetic variation for resistance to a specific pathogen within commercially relevant salmonid populations.
A long-term approach
Selective breeding is a long-term approach, regardless of the trait, for making improvements in productivity. Generally small-to-moderate improvements are made each generation, but importantly these small-to-moderate gains are cumulative and permanent in the population. For scientists or breeders that are interested in developing a selective breeding program for disease resistance, there are eight key considerations:
- Is it a high-priority disease for which current control strategies are either inadequate, not cost effective, or not sustainable?
- Has the disease-causing pathogen been isolated and can it be artificially propagated?
- Is there an existing high-throughput, repeatable disease challenge model? If not, can one be developed?
- Are pedigrees maintained for the population, either through individual rearing of families as small fry or by genotyping to reconstruct pedigrees? Regardless of the method, some means of tagging or marking replacement broodstock based on family of origin is essential.
- Is there sufficient genetic variation for disease resistance in the target population? How much genetic improvement is expected each generation, and at what “cost” to inbreeding accumulation?
- Will the improved resistance measured in the laboratory translate to improved resistance on the farm where fish are naturally exposed to the pathogen?
- Is there genetic diversity of the pathogen at the farm site(s)? How will this pathogen diversity impact resistance on the farm?
- Will selection for resistance to a specific pathogen result in a correlated response – either favorable or unfavorable – with other economically-important production traits?
Trout research at the NCCCWA
The USDA, ARS, National Center for Cool and Cold Water Aquaculture (NCCCWA; Leetown, W.V., USA) opened in 2001, and at that time NCCCWA scientists began developing a fully-pedigreed rainbow trout resource population and identifying high-priority diseases in domestic rainbow trout aquaculture.
Bacterial Cold Water Disease (BCWD), or rainbow trout fry syndrome, was identified as a high-priority disease affecting domestic rainbow trout aquaculture, and current disease control options were limited to management of culture conditions and antibiotic treatment. Fortunately, industry and academic colleagues had previously isolated, cultured, and identified the yellow-pigmented, gram-negative bacterium Flavobacterium psychrophilumas the causative agent of BCWD, tested various injection- and immersion-based laboratory challenge models, and provided evidence that resistance to this pathogen might be heritable in rainbow trout populations.
NCCCWA scientists obtained a single isolate of F. psychrophilum from a field outbreak, produced a large bank of cryopreserved stock for reproducible challenge, and began refining the injection-based (intraperitoneal) laboratory challenge model and developing the infrastructure to conduct high-throughput disease resistance testing. Thus, in 2005, with all the “pieces” in place, 71 full-sib families from the newly-developed resource population were tested for BCWD resistance in a single, large laboratory challenge, and improving BCWD resistance became the breeding objective for this population.
From this point on, the population became closed; that is, no outside germplasm was introduced into the population. For five generations (10 years), BCWD resistance remained the sole breeding objective, but the amount of selection pressure put on the population was constrained to limit the amount of inbreeding accumulation to not more than ~1 percent per generation.
Although not practical for an industry breeding program, reference control (randomly-mated) and susceptible lines were also developed alongside the resistant line to empirically quantify the amount of selection response and to address fundamental questions related to the biology of host resistance.
Five generations of selection produced considerable phenotypic divergence (i.e., a survival difference >60 percentage points between the resistant and susceptible lines) and the genetic lines stably express the respective phenotypes during growth and after transport. Farm trials identified that even after only three generations of selection, the resistant line exhibited a significant survival advantage compared to the susceptible line or hatchery stocks reared either in adjacent or serial raceways. A trial in which the three genetic lines were combined into a single rearing unit, and line determined by DNA analysis, demonstrated that the resistant line had lower BCWD-related mortality and pathogen load following natural exposure.
These studies validated the utility of the injection-based challenge model for phenotypic evaluation and suggest that performance improvement can occur after relatively few generations of breeding. Presumably the benefits of selection occur both through the concentration of resistance alleles and elimination of fish that exhibit general immunodeficiency. A surprising finding was a modest positive genetic correlation with improved resistance against columnaris disease, caused by Flavobacterium columnare. Thus, cross protection can be achieved against genetically related pathogens.
We have not identified negative effects of selective breeding on either growth or production traits in grow-out trials to ~600 g or ~2.5 kg target sizes. However, an inherent limitation of the line is the susceptibility to infectious hematopoietic virus as demonstrated by both laboratory challenge and epizootics in two grow-out trials. Thus, prior to starting breeding efforts it is recommended to evaluate susceptibility to other important pathogens as this susceptibility has limited adoption of the line in locations where IHNV is endemic.
Perspectives
More than 10 years of research on selective breeding has generated data indicating selective breeding can create considerable phenotypic divergence in rainbow trout lines when targeting a specific pathogen. And today’s breeder now has rapidly-evolving and increasingly-affordable genomic tools and technologies at their disposal and genomic selection has the potential to markedly expedite genetic improvement compared to traditional, family-based selective breeding.
On-farm benefits have been demonstrated and improved survival is a stable trait that is manifested as small as 0.2 grams and has life-time duration. The specificity of genetic disease resistance remains to be determined and it is not known if F. psychrophilum strains will evolve on-farm to circumvent breeding progress.
Research is ongoing to determine the genetic mechanisms of disease resistance as well as the impact of selective breeding on the immune response to other pathogens and commonly used vaccines. These efforts will be important to develop more specific and effective breeding aims, and to better inform vaccine development.
Our take-home message is that selective breeding is an important and effective tool – but not necessarily a silver bullet – that can complement good biosecurity, management and vaccination strategies to maintain healthy and productive populations.
Now that you've finished reading the article ...
… we hope you’ll consider supporting our mission to document the evolution of the global aquaculture industry and share our vast network of contributors’ expansive knowledge every week.
By becoming a Global Seafood Alliance member, you’re ensuring that all of the pre-competitive work we do through member benefits, resources and events can continue. Individual membership costs just $50 a year. GSA individual and corporate members receive complimentary access to a series of GOAL virtual events beginning in April. Join now.
Not a GSA member? Join us.
Authors
-
Timothy D. Leeds, Ph.D.
Research Geneticist
National Center for Cool and Cold Water Aquaculture
Agricultural Research Service, USDA
11861 Leetown Rd.
Kearneysville, WV 25430[118,111,103,46,97,100,115,117,46,115,114,97,64,115,100,101,101,108,46,109,105,116]
-
Gregory D. Wiens, Ph.D.
Molecular Biologist (Immunology)
National Center for Cool and Cold Water Aquaculture
Agricultural Research Service, USDA
11861 Leetown Rd.
Kearneysville, WV 25430
[118,111,103,46,97,100,115,117,46,115,114,97,64,115,110,101,105,119,46,103,101,114,103,65,83,85]
Tagged With
Related Posts

Health & Welfare
10 paths to low productivity and profitability with tilapia in sub-Saharan Africa
Tilapia culture in sub-Saharan Africa suffers from low productivity and profitability. A comprehensive management approach is needed to address the root causes.

Health & Welfare
Barcoding, nucleic acid sequencing are powerful resources for aquaculture
DNA barcoding and nucleic acid sequencing technologies are important tools to build and maintain an identification library of aquacultured and other aquatic species that is accessible online for the scientific, commercial and regulatory communities.

Innovation & Investment
AquaGen CEO: Genomics are transforming aquaculture
The CEO of AquaGen knew that the Norwegian research group’s work in genomics was key to the salmon industry’s future. And that was before she even worked there.

Health & Welfare
Born in Hawaii, SPF broodstock shrimp industry faces globalization
The next step for shrimp breeding will be developing animals that aren’t just disease-free, but increasingly resistant to multiple pathogens. The industry is globalizing, with suppliers setting up shop overseas. But its birthplace will always be Hawaii.