Dissolved nutrient essential for chlorophyll synthesis
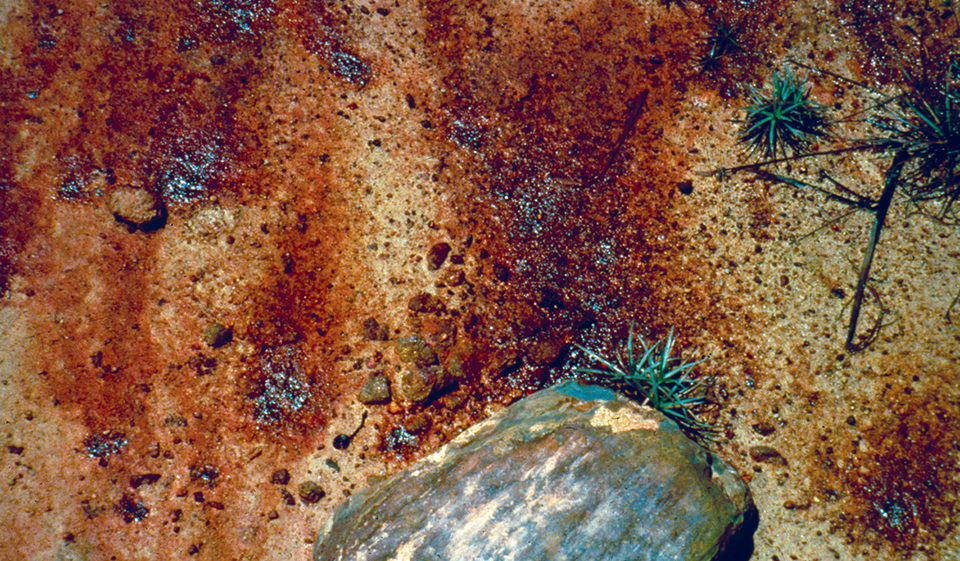
Iron is an important water quality variable in aquaculture. It is a dissolved nutrient required in small quantities by both aquatic plants and animals. But chemical reactions of iron in sediment and water can have negative impacts on aquatic life.
Varied concentrations
Iron occurs in the earth’s crust primarily as hydroxides and oxides such as ferric hydroxide and hematite. Most iron-bearing minerals are not very water soluble. The solubility of ferric hydroxide in distilled water at 25 degrees-C and pH 7, for example, is less than 1 part per trillion. Other iron minerals are even less soluble.
Such small concentrations of iron are too low to support the growth of plants. It is therefore fortunate that ferric iron reacts with major anions in water to form soluble ion pairs and complexes, and combines with dissolved organic matter to form soluble iron chelates. These combined forms of iron greatly increase the iron concentration in water and can be used by plants.
Typical iron concentrations in surface freshwater and the pore water of terrestrial soil and aquatic sediment are 50 to 100 parts per billion (ppb), levels that are adequate for plant growth. Waters with high concentrations of humic substances can have 1 to 10 parts per million (ppm) total iron. Plants typically contain 100 to 200 ppm iron on a dry matter basis.
Iron is essential for chlorophyll synthesis and a component of hemoproteins such as dehydrogenases and ferredoxins. Animals usually obtain iron from the food they eat and typically contain 100 to 500 ppm of this element on a dry-weight basis. In fish and other vertebrates, iron is a component of hemoglobin, which increases the oxygen-carrying capacity of blood. Iron also is important in hemoproteins that catalyze biochemical reactions in animals.
Although low iron concentration seldom limits the growth of phytoplankton in freshwater aquaculture ponds, iron limitation of phytoplankton can occur in some weakly mineralized waters without appreciable concentrations of dissolved organic matter. Normal seawater contains only 10 ppb iron, and additions of iron can stimulate phytoplankton productivity in ocean water.
Uses in aquaculture
Traditional aquaculture pond fertilizers can be supplemented with about 0.05 percent iron to improve their effectiveness in promoting phytoplankton growth in brackishwater or seawater. Mineral sources of iron such as ferrous sulfate and ferrous oxide are less expensive sources of iron for fertilizers, but chelated iron compounds should be used to enhance iron solubility and fertilizer effectiveness.
The solubility of iron minerals in soil and sediment increases as pH decreases. For example, the solubility of ferric iron from ferric hydroxide is about 1 million times greater at pH 5 than pH 7. Total soluble iron concentrations are much higher in acidic soils, sediments and waters than in neutral or alkaline ones. Iron solubility also increases as the redox potential or degree of oxidation declines.
In pond bottoms, the surface of sediment is oxidized because of contact with oxygenated water. Bacterial activity in sediment consumes dissolved oxygen from pore water faster than it can move downward through the pore space. Below a depth of a few millimeters, pond sediment usually is devoid of oxygen and has a low redox potential.
Ferric iron is changed to ferrous iron in the absence of oxygen in sediment. Ferrous compounds impart a dark color to sediment, and surface aerobic sediment usually is lighter in color than underlying, anaerobic sediment.
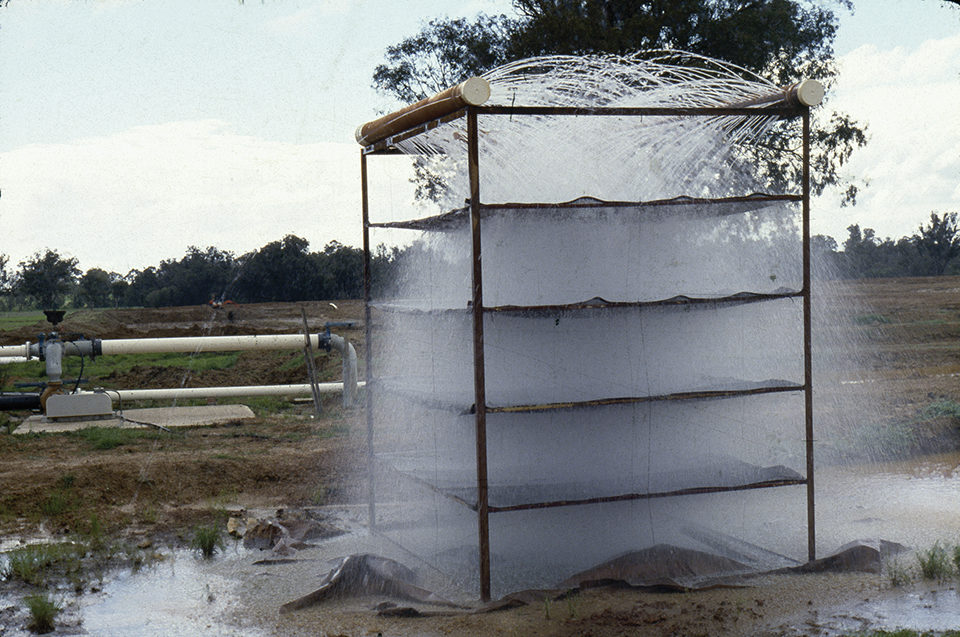
Pond bottoms
Ferrous iron in sediment often has a beneficial effect. Hydrogen sulfide is produced by microbial activity in reduced sediment, and this potentially toxic gas can diffuse into pond water. If ferrous iron is abundant in sediment pore water, it precipitates hydrogen sulfide as insoluble ferric sulfide (iron pyrite).
Hydrogen sulfide toxicity is not likely to occur in shrimp ponds where bottoms were constructed of soil with a high iron content. Treatment of pond sediment with iron slag, ferrous sulfate or ferrous oxide has been used as a hydrogen sulfide control technique.
Ferrous iron in pond soil is oxidized to ferric form during the dryout period between crops. The coloration of the soil can be used to follow the progress of soil oxidation.
Oxidation of large amounts of ferrous iron to ferric iron often results in reddish deposits of ferric hydroxide on pond bottoms. This phenomenon is particularly common where water seeps out of the embankments of empty ponds. After refilling, the water in such ponds may contain a reddish suspension of ferric hydroxide for a few days. The suspended particles should be allowed to settle before stocking ponds with fish or shrimp.
In ponds with acidic sediment, iron deposits can form on the exoskeletons and gills of shrimp. This can cause physical damage to the gills and unsightly blotches on shrimp at harvest. The most effective way of reducing iron concentrations in sediment pore water is application of agricultural limestone or lime to raise the pH.
Phosphate reacts with ferric iron to form relatively insoluble iron phosphate. However, when the redox potential declines, ferric iron is reduced to ferrous iron, releasing phosphate. Pore water of anaerobic sediment and oxygen-depleted pond water can have high concentrations of dissolved phosphate. Oxygenation of anaerobic water results in reprecipitation of ferrous iron as ferric hydroxide, and phosphate is adsorbed by the precipitating ferric hydroxide and removed from the water column.
Dissolved phosphate concentrations often increase in the bottom layers of ponds or lakes that thermally stratify in summer. In autumn, these water bodies destratify, and the bottom water is mixed with oxygenated water in upper layers with resulting reprecipitation of phosphate. Nevertheless, the increase in phosphorus concentration in surface water for a brief period following the overturn often triggers a temporary phytoplankton bloom.
Toxicity, measurement
Ground waters usually are depleted of dissolved oxygen, and well water can have high concentrations of ferrous iron. When such water is exposed to air, ferrous iron is oxidized and precipitates. This process can cause brownish or reddish stains on objects in contact with the water. In some situations, slimy mats of iron-oxidizing bacteria develop where wells discharge onto the land surface.
Iron is not directly toxic to organisms, and the precipitation of iron from well water entering ponds usually has no negative impacts. In hatcheries, however, precipitation of ferric hydroxide from well water can coat eggs or cause mechanical obstruction of the gills of fish. Well water that contains much ferrous iron can be treated by gravity aeration or mechanical aeration. The resulting ferric hydroxide can be removed by sedimentation, but a settling time of one or two days may be necessary. Alternatively, the iron can be quickly removed by sand filtration.
Measurement of iron concentrations in pond soils and waters usually is not necessary. Acidic soils should be limed irrespective of iron content, and raising the pH by liming will reduce the solubility of iron. High concentrations of iron in well water can be detected by placing some of the water in a clear container and aerating it by stirring. Precipitation occurs quickly, and the amount of the precipitate will be proportional to the ferrous iron concentration. The dark color caused by ferrous iron also allows one to detect anaerobic zones in sediment.
(Editor’s Note: This article was originally published in the May/June 2008 print edition of the Global Aquaculture Advocate.)
Now that you've finished reading the article ...
… we hope you’ll consider supporting our mission to document the evolution of the global aquaculture industry and share our vast network of contributors’ expansive knowledge every week.
By becoming a Global Seafood Alliance member, you’re ensuring that all of the pre-competitive work we do through member benefits, resources and events can continue. Individual membership costs just $50 a year. GSA individual and corporate members receive complimentary access to a series of GOAL virtual events beginning in April. Join now.
Not a GSA member? Join us.
Author
-
Claude E. Boyd, Ph.D.
Department of Fisheries and Allied Aquacultures
Auburn University
Auburn, Alabama 36849 USA[117,100,101,46,110,114,117,98,117,97,64,49,101,99,100,121,111,98]
Tagged With
Related Posts

Responsibility
A look at unit processes in RAS systems
The ability to maintain adequate oxygen levels can be a limiting factor in carrying capacities for RAS. The amount of oxygen required is largely dictated by the feed rate and length of time waste solids remain within the systems.

Health & Welfare
A holistic management approach to EMS
Early Mortality Syndrome has devastated farmed shrimp in Asia and Latin America. With better understanding of the pathogen and the development and improvement of novel strategies, shrimp farmers are now able to better manage the disease.

Responsibility
Addressing safety in Latin America’s tilapia supply chain
Over the last decade, the experience gained by many tilapia farmers combined with proficient programs implemented by local governments have significantly improved tilapia production in various Latin American countries like Colombia, Mexico, Ecuador and other important tilapia producers in the region.

Innovation & Investment
Algae innovators aim to freeze out early-stage shrimp losses
A greenhouse in Belgium believes its innovative shrimp feed product, made from freeze-dried microalgae, packs the necessary nutrients for the crustacean’s most vulnerable life stage: the first three days of its life.